FROM:
American J Clinical Nutrition 2012 (Feb); 95 (2): 428–436 ~ FULL TEXT
Cintia LN Rodacki, André LF Rodacki, Gleber Pereira, Katya Naliwaiko,
Isabela Coelho, Daniele Pequito, and Luiz Cléudio Fernandes
Paraná Federal University,
Setor de Ciências Biológicas,
Curitiba, Paraná, Brazil.
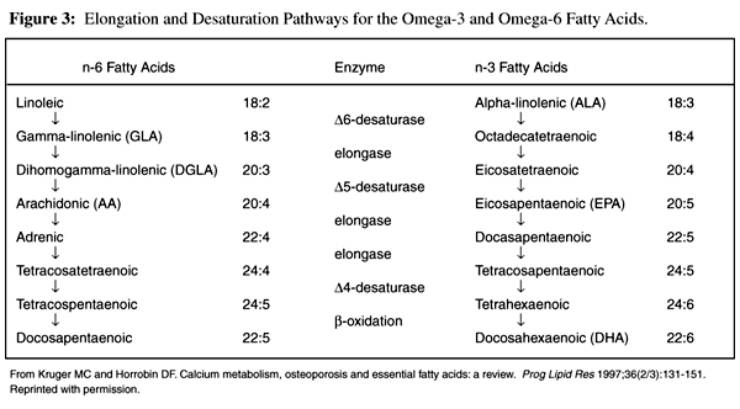
BACKGROUND: Muscle force and functional capacity generally decrease with aging in the older population, although this effect can be reversed, attenuated, or both through strength training. Fish oil (FO), which is rich in n-3 (omega-3) polyunsaturated fatty acids (PUFAs), has been shown to play a role in the plasma membrane and cell function of muscles, which may enhance the benefits of training. The effect of strength training and FO supplementation on the neuromuscular system of the elderly has not been investigated.
OBJECTIVE: The objective was to investigate the chronic effect of FO supplementation and strength training on the neuromuscular system (muscle strength and functional capacity) of older women.
DESIGN: Forty-five women (aged 64 ± 1.4 y) were randomly assigned to 3 groups. One group performed strength training only (ST group) for 90 d, whereas the others performed the same strength-training program and received FO supplementation (2 g/d) for 90 d (ST90 group) or for 150 d (ST150 group; supplemented 60 d before training). Muscle strength and functional capacity were assessed before and after the training period.
RESULTS: No differences in the pretraining period were found between groups for any of the variables. The peak torque and rate of torque development for all muscles (knee flexor and extensor, plantar and dorsiflexor) increased from pre- to posttraining in all groups. However, the effect was greater in the ST90 and ST150 groups than in the ST group. The activation level and electromechanical delay of the muscles changed from pre- to posttraining only for the ST90 and ST150 groups. Chair-rising performance in the FO groups was higher than in the ST group.
CONCLUSIONS: Strength training increased muscle strength in elderly women. The inclusion of FO supplementation caused greater improvements in muscle strength and functional capacity.
From the FULL TEXT Article:
INTRODUCTION
The ability of skeletal muscles to generate torque and the rate at which this torque is developed are reduced by aging. [1] Such a phenomena occurs due to changes in the neuromuscular system, including morphologic and phenotypic changes, change in size of muscle fibers, and loss of muscle mass [2]; neural degenerative disorders of the central and/or peripheral nervous system [3]; low physical activity level; and nutritional changes. [4] Therefore, different strategies to attenuate or reverse the decline of the neuromuscular system in the elderly are advised. Indeed, it has been shown that strength training is able to attenuate and/or partially reverse the functional capacity reduction of the neuromuscular system ability in the elderly. [5]
Some studies report that strength training increases peak torque and RTD [4–6], as well as functional capacity. [7] In addition, the neural excitability is improved after a strength-training program, which decreases the EMD—ie, the time between the electrical stimulation and the mechanical response of muscle. [8] This suggests that strength training can induce neuromuscular adjustment in older individuals, leading to improvements in the quality of life.
Western diets are high in n–6 and low in n–3 (omega-3) polyunsaturated fatty acids (PUFAs), which compete for incorporation into the labile second position of phospholipids in the cell membranes. n–6 PUFAs, mainly linoleic acid, is a precursor of arachidonic acid, which is a substrate of cyclooxygenase and lipoxygenase enzymes that leads to a net proinflammatory environment that will cause damage in several tissues, including muscles. On the other hand, n–3 PUFAs exert pleiotropic actions on many tissues, particularly in the cardiovascular and central nervous systems, that might be protective against age-related diseases. The central nervous system has a high concentration of complex lipids, which determine the structural and functional properties of its cellular and subcellular membranes. [9–11] FO supplementation, which is rich in n–3 PUFAs, has been shown to increase nerve conduction velocity in the elderly [12], sarcolemma ion channel modulation [13], and improved heart contractile activity. [14] Thus, because FO supplementation improves both cardiac muscle contractility [15] and nerve conduction velocity, it is reasonable to hypothesize that it may potentiate strength-training effects on skeletal muscles. There is a dearth of studies that report the effects of FO supplementation on neuromuscular function in the elderly and a lack of information regarding the length of supplementation and dose.
This study aimed to investigate the effect of FO supplementation associated with a strength-training program on the neuromuscular system (muscle strength and functional capacity) in elderly women. In addition, it also investigated whether the duration of FO supplementation modifies the effect. It was hypothesized that FO supplementation associated with strength training would potentiate neuromuscular function.
SUBJECTS AND METHODS
Participants
Table 1
|
Forty-five healthy white women (aged 64 ± 1.4 y) volunteered to participate in this study (Table 1). They were screened by a physician and were deemed in good health to perform strength training. The participants were neither receiving hormone replacement therapy nor engaged in any other regular physical activity program during the 12 mo before the present study. Positivo University's ethics committee granted approval for the experimental procedures, and participants signed an informed consent form before starting the procedures. The study commenced in March 2008.
In the first session, participants answered the International Physical Activity Questionnaire [16], and no differences in physical activity level were identified between groups. In an attempt to obtain 3 homogeneous groups, participants were randomly assigned to 1 of the 3 experimental groups, after being classified in quartiles according to their relative knee extension strength (one repetition maximal/body weight). [5] One group performed only the strength training (ST group; n = 15). The ST90 group (n = 15) was supplemented with FO throughout the 90 d of strength training. Finally, the ST150 group (n = 15) started the FO supplementation 60 d before commencing the strength training. The FO supplement contained 180 g EPA and 120 g DHA/kg. All participants in the supplemented groups received 2 g/d of FO (one capsule during each main meal), which provided ~ 0.4 g EPA and 0.3 g DHA/d.
The participants were asked to maintain their normal eating habits and physical activity level throughout the study (Table 1). The strength-training program was designed to improve lower limb muscle strength. The program included supervised resistance-training exercises performed 3 times/wk, for 12 wk, for a total of 36 training sessions. Diet was monitored by using food-frequency questionnaires completed before and after training to ensure that there was no change in dietary intake. Neuromuscular (first session) and functional capacity (second session) assessments were performed before (pre–) and after (post–) the training period for the ST and ST90 groups. The ST150 group participants were evaluated at 3 different times: presupplementation (base, collected 60 d before initiating pretraining period), pretraining (at 60th day to coincide with pre–), and posttraining (at 150th day to coincide with post–). Special care was taken so that the participants involved in this study did not come into contact with other groups during training or blood collection to minimize the chances of psychological effects. In addition, the ST group trained on different days of the week (ie, Tuesdays, Thursdays, and Saturdays) from the supplemented groups (ST90 and ST150; ie, Mondays, Wednesdays, and Fridays). The participants received specially worded consent forms to ensure that they were unaware of the existence of other experimental groups. A placebo was not provided because 1) there was no contact between groups and 2) there was no suitable placebo available to the experimenters for FO supplementation. Providing any other oil could cause its incorporation into the cell membranes and increase oil consumption, which has been associated with the development of chronic diseases. [17] All participants concluded the experimental procedures.
Fatty acid composition of plasma and of the FO capsule
The fatty acid composition of the FO capsules as well of the plasma of the participants was assessed by HPLC (Varian ProStar). Total lipids were extracted from the FO capsules and plasma of volunteers by using chloroform-methanol (2:1 vol:vol). Fatty acids were derivatized with 4–bromomethyl-7-coumarin and then separated by using an octadecylsilica column (25 cm × 4.6 mm internal diameter; particle size: 5 µm). Fatty acids were resolved isocratically by using a mobile phase of acetonitrile-water (gradient from 77:23 to 90:10 vol:vol) at a flow rate of 1 mL/min. Fatty acid derivatives were detected by fluorescence (325–nm excitation, 395–nm emission), as previously described. [18] The analysis showed the presence of the following fatty acids in the capsules provided to the participants (mean ± SD): lauric (12:0), 7.0 ± 0.1%; miristic (14:0), amount not determined; palmitic (16:0), 14.2 ± 1.9%; stearic (18:0), 1.4 ± 0.4%; palmitoleic (16:1n–7), amount not determined; oleic (18:1n–9), 10.6 ± 1.1%; linoleic (18:2n–6), 3.3 ± 0.3%; α-linolenic (18:3n–3), amount not determined; arachidonic (20:4n–6), 13.7 ± 0.8%; EPA (22:5n–3), 29.5 ± 0.7%; DHA (22:6n–3), 23.6 ± 0.2%.
Strength-training program
After warming up, a 1RM was performed in the first training session. The participants were familiarized with the strength-training exercises for 6 sessions (2 wk) by performing 3 sets of 8 repetitions at 50% of their 1RM load. After the familiarization period, 12 wk of strength training were introduced. The first training week included 3 sets of 8 repetitions at 70% of 1RM. Training load was increased in the second week to 80% of 1RM and was adjusted on a weekly basis (every 3 sessions) from the third to the last week of training. Similar procedures have been used in previous studies of muscle strength in the elderly. [19] The sessions were performed under the supervision of one researcher and under the guidance of 2 qualified instructors.
The following exercises were performed: bilateral knee flexion and extension, bilateral hip adduction and abduction, unilateral hip extension and flexion, bilateral horizontal leg press, and bilateral plantar flexion. Exercises were performed that included concentric and eccentric phases using pulley-type machines (Athletic). Verbal encouragement was provided during each exercise series. The load for each exercise was recorded throughout the experimental period to quantify load improvements.
Peak torque and RTD
Maximal voluntary isometric contraction was used to determine the peak torque, defined as the best performance from 3 trials. It was calculated by multiplying the force, measured by a strain gauge (model CZC500; Kratos), by the distance from its attachment point to the segment joint center. The lead (steel cable) of the strain gauge was firmly secured on the distal portion of the dominant segment by an adjustable Velcro strap. [5, 20] One minute of rest was allowed between each trial. The participants were asked to exert a maximum effort (ie, to push as hard and fast as possible) with the dominant segment positioned at ~ 90° (knee flexion, knee extension, and dorsi- and plantar flexion). From the maximal voluntary isometric contraction, the RTD was calculated by using a customized routine (Matlab 6.0; MathWorks, Inc) and defined as the slope of the force-time curve determined between 20% and 80% of the peak torque value. [20]
Muscle activation and EMD
A surface electromyography device (model Telemyo 900; Noraxon) was used to evaluate the muscle activation and EMD during the maximal voluntary isometric contractions. The electromyography of the rectus femoris, vastus lateralis, biceps femoris, tibialis anterioris, gastrocnemius, and soleus muscles were recorded synchronically with force traces by using a common pulse marker. The electrodes were placed with an interelectrode distance of 1.5 cm on the largest muscle bulk, following the orientation of muscle fibers. To ensure the same placement of electrodes pre– and posttraining, a transparent plastic sheet was used to identify the exact electrode sites in relation to anatomic points, scars, and marks on the skin. The acquisition frequency of the electromyography was set at 1000 Hz, and band pass filters with cutoff frequencies of 20 and 500 Hz were applied to the raw data. The muscle activation level was determined by calculating the RMS, 500 ms around the peak torque, with the use of specific software (model Telemyo 900; Noraxon). The EMD was determined by the time lag between the beginning of the electromyographic activity and the beginning of the mechanical response (torque increase). The beginning of the electromyographic activity was determined as an increase in the muscle activation of >2 SDs from resting values. The initiation of torque was determined by the force trace increasing to 2 SDs from resting values.
Functional capacity
Functional capacity was determined in the second assessment session and included the following tests: “foot up and go,” “sit and reach,” “chair rising,” and 6–min walk. Tests were performed with an interval of 20 min between each and are described in detail elsewhere. [21, 22]
Statistical analysis
After confirming data normality and variance homogeneity, a number of analyses of variance (2–factor ANOVA), with group (ST, ST90, and ST150) and time (pre– and posttraining) as factors, were conducted with the last factor treated as a repeated measure. A dependent-sample t test was used for ST150 group data to compare the dependent variables before the supplementation period (baseline) with the pretest data (pretest), and no significant differences were observed. Hence, the baseline data of the ST150 group were not emphasized in the analysis. The EMD data were analyzed by using an ANCOVA, with the pretraining data as a covariate and group as a factor. The Bonferroni-corrected t test was applied to identify where differences occurred. Statistical analyses were performed by using Statistica software (version 7.0; Statsoft Inc). The significance level was set at P < 0.05.
RESULTS
Body mass, BMI, and eating habits for all groups in the pre– and posttraining periods are shown in Table 1. Body mass and dietary habits remained unchanged from pre– to posttraining in all groups (P > 0.05). The total mean energy, carbohydrate, protein, and lipid intake were similar between groups (group effect; P > 0.05) and did not change after the training period (time effect; P > 0.05).
Table 2
|
Plasma lipid profiles for all groups are shown in Table 2. The ST group showed no change in EPA and DHA (P > 0.05). The ST90 group experienced significant increases (P < 0.05) in EPA and DHA concentrations in the plasma after FO supplementation (posttraining) of 45% and 20%, respectively. The ST150 group also showed significant mean increases in EPA and DHA concentrations in the plasma after FO supplementation (from baseline to pretraining) of 112% and 50%, respectively. No changes were observed in EPA and DHA in the ST150 group from pre– to posttraining (P > 0.05).
Peak torque and RTD
Figure 1
|
Peak torque values in the muscles analyzed pre– and posttraining in the ST, ST90, and ST150 groups are presented in Figure 1. There were no differences between groups in peak torque pretraining (P > 0.05). Peak torque in the knee flexor and extensor, plantar and dorsiflexor muscles increased from pre– to posttraining in all groups (time effect; P < 0.05). However, FO supplementation resulted in significantly higher peak torque posttraining than in the ST group for all muscles assessed (P < 0.05). A longer supplementation period (ST150) did not cause any additive effect, and results were similar to those for the ST90 group (P > 0.05) but higher than for the ST group (P < 0.05).
Figure 2
|
The RTDs in the muscles analyzed pre– and posttraining in the ST, ST90, and ST150 groups are presented in Figure 2. There were no differences in RTD pretraining between groups (P > 0.05). RTD in the knee flexor and extensor, plantar, and dorsiflexor muscles increased from pre– to posttraining in all groups (time effect; P < 0.05). However, FO supplementation resulted in significantly higher RTD posttraining than in the ST group for all muscles assessed (P < 0.05). A longer supplementation period (ST150) did not cause addictive effect, and results were similar to those for the ST90 group (P > 0.05) but were higher than for the ST group (P < 0.05).
Electromyography and EMD
Figure 3
|
The muscle activation level was determined by calculating the RMS for the muscles analyzed pre– and posttraining (Figure 3). Pretraining RMS values were similar between groups (P > 0.05). RMS increased from pre– to posttraining in all groups (P < 0.05 compared with pretraining). When FO supplementation was included, the RMS of the vastus lateralis, rectus femoris, biceps femoris, tibialis anterioris, and soleus in the ST90 and ST150 groups increased significantly when compared with the ST group (P-interaction < 0.05). Supplementation for a long period caused a change in the RMS only in the gastrocnemius muscle, which showed higher activity in the ST150 group than inthe ST90 group (P < 0.05).
Figure 4
|
The time lag between the beginning of the electromyographic activity and the onset of the mechanical response (EMD) is shown in Figure 4. There were no differences in EMD pretraining between groups (P > 0.05). EMD of rectus femoris and gastrocnemius muscles in the ST group decreased significantly from pre– to posttraining (P < 0.05). The inclusion of FO supplementation markedly reduced the EMD of all muscles from pre– to posttraining (time effect, P < 0.05). The FO–supplemented groups (ST90 and ST150) showed a significant EMD reduction when compared with the ST group (P < 0.05). No differences (P < 0.05) were found between the FO–supplemented groups (ST90 and ST150).
Functional capacity
Figure 5
|
Functional capacity was determined by “foot up and go,” “sit and reach,” “chair-rising,” and “6–min walk” tests (Figure 5). There were no differences between the groups pretraining in any of these measures (P > 0.05). Posttraining, the ST group showed improvement in the chair-rising test (P < 0.05). FO supplementation induced a further increase in the ST90 group (P < 0.05), but a longer supplementation period (ST150) did not further change functional capacity (P > 0.05 compared with ST90). There were no alterations in the sit-and-reach test after strength training or FO supplementation (P > 0.05 compared with pretest). The foot up and go test was performed significantly faster posttraining in all groups (P < 0.05 compared with pretest). There was a significant increase in 6–min-walk performance (P < 0.05) posttraining induced by exercise (ST group). The FO-supplemented groups (ST90 and ST150) showed similar results when compared with the ST group (P > 0.05) and did not differ between the 2 supplementation durations (P > 0.05).
DISCUSSION
The present study aimed to determine the effects on the neuromuscular system (ie, muscle strength and functional capacity) of FO supplementation when used in addition to a strength-training program in elderly women and to determine whether FO supplementation for a longer period causes further effects. The main finding was that FO supplementation along with strength training improved the response of the neuromuscular system. However, supplementation with FO for an additional period pretraining did not cause any additional effects.
Body weight remained unchanged among groups during the period of the study (Table 1). This indicated that the amount of FO provided (2 g/d) did not cause changes in body mass. Participants’ dietary habits, which remained unchanged for the duration of the study, may have also contributed to body weight stability because the amount of calories consumed was consistent across the dietary habit assessments. Thus, the stable dietary intake variations should not be seen as a confounding factor.
Strength training induced an increase in peak torque in the elderly women who were tested; the strength gains reported in the present study were higher than those reported by others. For example, Ferri et al [23] showed increased knee extensor and plantar flexor peak torques of 19% and 12%, respectively, whereas Brown and Wheir [24] observed increases of 17% for the knee extensor muscles, which are lower than those reported in the present study. The larger gains observed in the present study (~ 50%) may be attributed to the participants’ lower initial muscle fitness, because most participants were sedentary at baseline. The high adherence to the training program may have also positively influenced the results. In addition, the continuous control of the training loads (adjusted weekly) may have played a role. Regardless of the factors that mediated such gains, the strength-training effects on the neuromuscular system corroborate other studies.
The improved RTD reflects improved muscle contractility, which is an important measure for the elderly due to its contribution to several daily life tasks [20] and its relation to the ability to recover from a slip or trip, preventing a fall. Strength training has been shown to facilitate muscle protein anabolism, energy expenditure, and improved appetite and food intake in elderly people. [25] Smith et al [26] recently reported that long-chain n–3 polyunsaturated fatty acids (PUFAs) have anabolic properties in healthy young and middle-aged adults. Current results suggest that the same is true in our more senior population.
When FO supplementation was associated with strength training there was an additional increase in peak torque and RTD. This study is the first, to our knowledge, to show that FO supplementation is able to enhance skeletal muscle activation and force when incorporated with strength training in elderly women. Indeed, concentrations of EPA (20:5n–3) and DHA (22:6n–3) in the plasma of FO-supplemented groups (ST90 and ST150) increased and indicated the incorporation of these fatty acids in the cells, particularly in the nervous system and muscles. The uptake of fatty acids occurs from VLDL and, in the tissues, involves several isoforms of fatty acid transporter. DHA is taken up and retained in the nervous system; once in the cell membrane and in other organelles, 20–carbon fatty acids originate eicosanoids, whereas DHA produces docosanoids (neuroprostanes, maresins, neuroprotectin D1, and related 22–carbon derivatives). n–3 PUFAs can stimulate the mitogen-activated protein kinase pathway and facilitate channel opening and gene expression due to their transcription regulator function. The investigation of the role of docosanoids and the existence of DHA-specific fatty acid receptors in the skeletal muscle tissues and neuromuscular system would be an interesting topic for future research. [27]
Several mechanisms are involved in muscle strength improvement. Initial gains may be attributed to the neural adjustment that occurs during the first weeks of training, which are followed by enlargement in muscle cross-sectional area. [28] With regard to neural adaptation (ie, muscle activation measured by electromyography), the analyzed muscle groups showed greater activation (electromyography) when FO supplementation was provided (Figure 3). One possible physiologic mechanism is that the FO may improve muscle function, changing the fluidity of the membrane and acetylcholine sensitivity. Patten et al [29] observed changes in several pharmacologic agents, such as acetylcholine, 8–iso-prostaglandins series 2, PGE2, and F2 and the thromboxane mimetic U-46619, which occurred in response to FO supplementation and which may have mediated contractility increases. Acetylcholine, iso-prostaglandins, and thromboxanes may act via G-protein–coupled receptor systems on the cell membrane that regulate cellular function. [29] It is well established that acetylcholine is an excitatory neurotransmitter that triggers the muscle contraction process. Thus, it may assist in muscle contraction by facilitating fast synaptic transmission at the neuromuscular junction (excitatory potential action) and elicit faster muscle contraction. Moreover, changes in the lipid composition of neural membranes affect endocytosis, exocytosis, membrane fusion, neurotransmitter uptake and release, and the activities of membrane-associated enzymes. [30] Better membrane fluidity and faster conduction velocity may facilitate impulse transmission. [31, 32] Such neuronal adaptations, due to FO supplementation, may then explain not only the electromyographic activation level improvements but also the EMD reduction, although there was a large within-group variation in the initial EMD condition.
Improvements in functional capacity after training showed that physical activity is an important component to improving quality of life. The similarities in the flexibility (sit-and-reach test) found in all groups can be explained by the lack of a specific stretching stimulus during the training period. The chair-rising test was the only functional test that was influenced by FO supplementation. Bassey et al [35], using very elderly participants (age >85 y), found a positive correlation between leg extensor strength and chair rising, suggesting that strength and RTD improvements may influence functional capacity. [36] The changes in peak torque and RTD observed around the knee joint for the ST150 group suggest that improvements in functional capacity may be viewed as a direct response to improved muscle performance.
As far as we are aware, there have been no studies investigating the effect of length of FO supplementation on the neuromuscular system. In the present study, 90 d of FO supplementation potentiated the effects of an equal length of strength training, although the dose provided was below that recommended by the American Heart Association and the UK government. However, the inclusion of an additional period of 60 d of FO supplementation did not produce further effects. This indicates that the dose provided during 3 mo was sufficient to induce important physiologic changes in the neuromuscular system. These results should be viewed with caution because the participant numbers were relatively small. Other studies involving a larger sample and other combinations of training and supplementation period are required.
In conclusion, the use of FO supplementation in addition to strength training potentiates the neuromuscular system, enhancing the muscle strength and the functional capacity in elderly women. Thus, FO may be an attractive supplement for the elderly to maximize their neuromuscular responses to strength training, which is important to life quality. However, the supplementation length does not influence the strength-training effects.
Acknowledgments
We thank Neil Edward Fowler, from the Manchester Metropolitan University, United Kingdom, for his help with the final version of the manuscript.
The authors’ responsibilities were as follows—All authors: contributed substantially to the manuscript, which not only involved the work to conduct the strength-training program (CLNR, ALFR, GP) but also involved extensive time in laboratory analyses (CLNR, ALFR, DP, IC); CLNR, DP, IC, KN, LCF: contributed to data collection and to conducting the training processes; CLNR, ALFR, GP, DP, IC, KN, LCF: contributed to the final version of the manuscript, for which they provided relevant intellectual contribution. This study involved integrative research that used different backgrounds and areas of expertise. There were no conflicts of interest for any of the authors.
Abbreviations used:
EMD = electromechanical delay
FO = fish oil
RMS = root mean square
RTD = rate of torque development
ST group = strength-training group
ST90 group = strength-training group with 90 d of FO supplementation
ST150 group = strength-training group with 150 d of FO supplementation
1RM = one-repetition maximal test.
References:
Hughes VA, Frontera WR, Wood M, Evans WJ, Dallal GE, Roubenoff R, Fiatarone Singh MA.
Longitudinal muscle strength changes in older adults: influence of muscle mass, physical activity, and health.
J Gerontol Biol Sci 2001;56A:B209–17
Lexell J, Taylor CC, Sjostrom M.
What is the cause of the ageing atrophy?
J Neurol Sci 1988;84:275–94
Scaglioni G, Narci MV, Mafiuletti NA, Pensini M, Martin A.
Effect of ageing on the electrical and mechanical properties of human slues motor unit activated by the H reflex and M wave.
J Physiol 2003;548:649–61
Kim JS, Wilson JM, Lee SR.
Dietary implications on mechanisms of sarcopenia: roles of protein, amino acids and antioxidants.
J Nutr Biochem 2010;21:1–13
Persch LN, Ugrinowitsch C, Pereira G, Rodacki ALF.
Strength training improves fall-related gait kinematics in the elderly: a randomized controlled trial.
Clin Biomech (Bristol, Avon) 2009;24:819–25
Narici MV, Hoppeler H, Kayser B, Landoni L, Claassen H, Gavardi C, Conti M, Cerretelli P.
Human quadriceps cross-sectional area, torque and neural activation during 6 months training strength.
Acta Physiol Scand 1996;157:175–86
Miszko TA, Cress ME, Slade JM, Covey CJ, Agrawal SK, Doerr CE.
Effect of strength and power training on physical function in community-dwelling.
J Gerontol 2003;58:171–5
Grosset JF, Piscione J, Lambertz D, Pérot C.
Paired changes in electromechanical delay and musculo-tendinous stiffness after endurance or plyometric training.
Eur J Appl Physiol 2009;105:131–9
Yehuda S, Rabinovitz S, Mostofsky DI.
Essential fatty acids are mediators of brain biochemistry and cognitive functions.
J Neurosci Res 1999;56:565–70
Gerbi A, Maixent JM, Ansaldi JL.
Fish oil supplementation prevents diabetes-induced nerve conduction velocity and neuroanatomical changes in rats.
J Nutr 1999;129:207–13
Rapoport SI.
In vivo fatty acid incorporation into brain phospholipids in relation to plasma availability, signal transduction and membrane remodeling.
J Mol Neurosci 2001;16:243–61
Johnson EJ, McDonald K, Caldarella SM, Chung HY, Troen AM, Snodderly DM.
Cognitive findings of an exploratory trial of docosahexaenoic acid and lutein supplementation in older women.
Nutr Neurosci 2008;11:75–83
Supari F, Ungerer T, Harisson DG.
Fish oil treatment decreased superoxide anions in the myocardium and coronary arteries of atherosclerotic monkeys.
Circulation 1995;91:1123–8
Holguin F, Tellez-Rojo MM, Lazo M, Mannino D, Schwartz J, Hernández M, Romieu I.
Cardiac autonomic changes associated with fish oil vs. soy oil supplementation in the elderly.
Chest 2005;4:1102–7
Egert S, Stehle P.
Impact of n–3 fat acids on endothelial function: results from human interventions studies.
Curr Opin Clin Nutr Metab Care 2011;14:121–31
Craig CL, Marshall AL, Sjostrom M, Bauman AE, Booth ML, Ainsworth BE, Pratt M, Ekelund U et al.
International physical activity questionnaire: 12-country reliability and validity.
Med Sci Sport Exerc 2003;35:1381–95
Simopoulos AP.
Evolutionary aspects of the diet omega-6:omega-3 fatty acid ratio: medical implications.
World Rev Nutr Diet 2009;100:1–21
Naliwaiko K, Fonseca RV, Castilho JC, Andreatini R, Bellissimo MI, Oliveira BH, Martins EF.
Effects of fish oil on the central nervous system: a new potential antidepressant?
Nutr Neurosci 2004;7:91–9
Flynn MG, Fahlman M, Braun WA, Lambert CP, Bouillon LE, Broslinsos PG, Armstrong CW.
Effects of resistance training on selected indexes of immune function in elderly women.
Am Physiol Soc 1999:1905–13
Bento PCB, Pereira G, Ugrinowitsch C, Rodacki ALF.
Peak torque and rate of torque development in elderly with and without fall history.
Clin Biomech (Bristol, Avon) 2010;25:450–4
Alves RV, Mota J, Costa MC, Alves JGB.
Physical fitness and elderly health effects of hydrogymnastics.
Rev Bras Med Esporte 2004;10:31–7
Misic MM, Valentine RJ, Rosengren KS, Woods JA, Evans EM.
Impact of training modality on strength and physical function in older adults.
Gerontol 2009;55:411–6
Ferri A, Scaglioni G, Pousson M, Capodaglio P, Van Hoecke J, Narici M.
Strength and power changes of the human plantar-flexion and knee extensors in response to resistance training in old age.
Acta Physiol Scand 2003;177:69–78
Brown L, Wheir J.
ASEP procedures recommendations I: accurate assessment of muscular strength and power.
J Exerc Physiol 2001;4:1–21
Volkert D.
The role of nutrition in the prevention of sarcopenia.
Wien Med Wochenschr 2011;161:409–15
Smith GI, Atherton P, Reeds DN, Mohammed BS, Rakin D, Rennie MJ, Mittendorfer B.
Omega-3 polyunsaturated fatty acids augment the muscle protein anabolic response to hyperinsulinemia-hyperaminoacidaemia in healthy young and middle-aged men and women.
Clin Sci (Lond) 2011;121:267–78
Bazan NG, Molina MF, Gordon WC.
Docosahexaenoic acid signalolipidomics in nutrition: neuroinflammation, macular degeneration, Alzheimer's and other neurodegenerative diseases.
Annu Rev Nutr 2011;31:321–51
Häkkinen K, Alen M, Kallinen M, Newton RU, Kraemer NU.
Neuromuscular adaptation during prolonged strength training, detraining and re-strength-training in middle-aged and elderly people.
Eur J Appl Physiol 2000;83:51–62
Patten GS, Abeywardena MY, Mcmurchie EJ, Jahangiri A.
Dietary fish oil increases acetylcholine- and eicosanoid-induced contractility of isolated rat ileum.
J Nutr 2002;132:2506–13
Farooqui AA, Horrocks LA.
Glycerophospholipids in the brain: phospholipases A2 in neurologic disorders
In: Farooqui AA, Horrocks LA eds.
Phospholipase A2-generated lipid.
New York, NY: Springer 2006: 115–24
Kinsella JE.
Effects of polyunsaturated fatty acids on factors related to cardiovascular disease.
Am J Cardiol 1987;60:23–32. MedlineGoogle Scholar
Zérouga M, Beauge F, Niel E, Durand G, Bourre JM.
Interactive effects of dietary (n–3) polyunsaturated fatty acids and chronic ethanol intoxication on synaptic membrane lipid composition and fluidity in rats.
Biochim Biophys Acta 1991;1086:295–304. MedlineGoogle Scholar
Bassey EJ, Fiatarone MA, O'Neill EF, Kelly M, Evans WJ, Lipsitz LA.
Leg extensor power and functional performance in very old men and women.
Clin Sci 1992;82:321–7
Skelton DA, Greig CA, Davies JM, Young A.
Strength, power and related functional ability of healthy people aged 65–89 years.
Age Ageing 1994;23:371–7
Return to OMEGA-3 FATTY ACIDS
Since 7–12–2017
|