FROM:
Functional Foods in Health and Disease 2014; 4 (7): 299–311 ~ FULL TEXT
David W. Lescheid, PhD, N.D.
David W Lescheid, PhD, N.D.,
Lichtentaler Strasse 48,
76530 Baden-Baden, Germany
A substantial and increasing body of clinical evidence supports the role of specific strains and mixtures of probiotics in the prevention and treatment of certain diseases. Several general mechanisms of action have been proposed, including supporting repair of hyperpermeable epithelial barriers, interfering with infection by pathogens, and restoring a healthful balance of commensal microbes to affect metabolism. Emerging evidence supports an additional role of probiotics as important modulators of immune system responses, including inflammation, at mucosal surfaces. In particular, by preventing or repairing ‘leaky’ epithelial barriers, probiotics can indirectly affect the inflammatory response by negating the source of pro-inflammatory stimuli associated with low-grade endotoxemia. They also enhance production of short chain fatty acids with anti-inflammatory properties (e.g. butyrate) as well as increase synthesis of antimicrobial peptides that influence inflammation resolution pathways in the mucosa. Furthermore, probiotics and some of their secreted metabolic products can act as ligands for innate immune system receptors, directly influencing key pro-inflammatory pathways. They also stimulate the differentiation and activity of important immune cells (e.g., dendritic cells, T cells), and subsequently increase production of important regulatory cytokines, including interleukin-10 (IL-10) and transforming growth factor-beta (TGF-β). Finally, there are limited but increasing animal studies and clinical trials demonstrating probiotics do affect common biomarkers of inflammation, including C-reactive protein, as well as signs and symptoms of the associated diseases suggesting they can have therapeutic benefit in the treatment of chronic inflammatory disease.
Keywords: probiotics, inflammation, endotoxemia, epithelial, cytokines, immune, butyrate, antimicrobial peptides
From the FULL TEXT Article:
INTRODUCTION
Our understanding of the beneficial roles of commensal microorganisms in sustaining human health has increased dramatically in recent times. It is evident a prolonged disruption in the normal ecology of commensal microflora (often termed dysbiosis) can contribute to different diseases through a number of mechanisms. For example, a reduced diversity of ‘healthy’ microbiota and subsequent opportunistic overgrowth of potentially pathogenic commensal bacteria and fungi alters signaling to the immune system. This results in abnormal activation of certain immune cells (e.g., Th1, Th17, gamma delta T (γδT) cells and innate lymphocytes) and repression of certain regulatory mechanisms (e.g., reduction of the activity and number of Treg and Tr1 cells, reduced immunoglobulin A (IgA) synthesis and lowered levels of major suppressive cytokines such as IL-10 and TGF-β) [1]. In general, the immunological outcome of dysbiosis is a shift away from the normal state of tolerance to an abnormal state of heightened reactivity towards commensal microbiota and dietary substances, resulting in chronic inflammation. There is a substantial and increasing body of scientific literature demonstrating that supplementation with probiotics can help treat dysbiosis, restore a healthy microbial ecology, and modulate the chronic inflammatory processes that can lead to disease.
REVIEW
Probiotics can help regulate inflammation in a number of ways, but this paper will focus primarily on:
1) indirect effects, by maintaining or repairing epithelial barriers, enhancing production of short chain fatty acids with anti-inflammatory properties (e.g., butyrate) as well as increasing synthesis of antimicrobial peptides that influence inflammation resolution pathways in the mucosa and
2) direct effects, by binding innate immune system receptors and triggering pathways that affect the production of cytokines associated with inflammation.
Indirect effects: 1) Repair of hyperpermeable epithelial barriers
A hyperpermeable epithelial barrier in the gastrointestinal tract is proposed as a major cause of chronic inflammation in a recently described pathophysiological process termed low-grade or subclinical endotoxemia. This is also termed metabolic endotoxemia if the cause(s) of the hyperpermeability are metabolic in origin, including a high-fat diet. In general, low-grade endotoxemia means there is abnormal translocation of macromolecules, including Gram-negative bacteria across the barrier, resulting in the lipopolysaccharide (LPS) (also termed endotoxin) portion of their cell wall, entering circulation to act as a constant low-level pro-inflammatory stimulus. This is in contrast to the most commonly recognized abnormal translocation of LPS, where a relatively large amount (>10-200 ng/ml) enters systemic circulation to cause overwhelming systemic inflammation and the serious and potentially fatal condition termed sepsis [2]. The medical consequences of this persistent ‘trickle’ of LPS (e.g., 1pg-100pg/ml) are potentially enormous with metabolic endotoxemia recently referred to as “one of the key culprits in provoking a non-resolving low-grade inflammation” [2]. Indeed, many different chronic diseases, including obesity [3], diabetes [4], fatty liver disease and nonalcoholic steatohepatitis [5], atherosclerosis, Parkinson’s disease [6], and cancer cachexia [7], are now correlated with metabolic endotoxemia.
Treatment of low-grade endotoxemia requires a comprehensive clinical approach that includes alteration of certain dietary choices and lifestyle behaviors, inclusion of medications with anti-inflammatory and/or drainage properties, but most importantly, repair of damaged epithelial barriers. A number of different natural health products will help repair a hyperpermeable epithelial barrier, including glutamine [8], quercetin, zinc [9] (e.g., zinc carnosine [10]) and different herbal medicines, but undoubtedly the most studied product is probiotics.
There are a number of mechanisms whereby certain probiotic strains can improve the structure and function of intestinal epithelial barriers, including buttressing the physical barrier, increasing mucin production, promoting production of antimicrobial peptides and heat shock proteins, attenuating the negative effect of pathogenic microorganisms, and modulating signaling pathways that affect cell proliferation and survival [11, 12]. For a diagrammatic representation of the various mechanisms of action of probiotics refer to [11, 12]. Of particular interest is the ability of specific probiotic strains to significantly decrease tumor necrosis factor alpha (TNF-α a pro-inflammatory cytokine that causes leaky barriers by directly impairing the integrity of the tight junctional complexes between epithelial cells. Other probiotic strains bolster mucosal membrane integrity by directly affecting expression of one or more of the important tight junctional proteins (e.g., zonulin-1, occludin and cingulin) [16, 17]. Various other cellular and molecular mechanisms (eg. release of metabolites and bioactive molecules, suppression of oxidative stress, interference with inflammatory pathways, increase expression of mucins, augment levels of IgA) are used by different probiotic strains to help protect and repair epithelial barriers [18, 19]. Several different probiotics including Lactobacillus species (eg. L. rhamnosus GG, L. plantarum DSM 9843, L reuteri R2LC, L casei DN-114001, L. paracasei NCC2461), Bifidobacterium infantis, Saccharomyces boulardii and S. cerevisae UFMG 905 and Escherichia coli Nissle 1917 have been shown to protect and repair epithelial barriers in vitro [18, 19].
There also are a number of animal studies [20, 21] and clinical trials [22, 23, 24, 25, 26, 27] demonstrating the efficacy of different probiotics in repairing or preventing damage to epithelial barriers caused by different diseases or other stressors.
Indirect effects: 2) Increase production of short chain fatty acids including butyrate
Another indirect effect of probiotics on inflammation occurs via their ability to ferment certain types of fiber, thus increasing the production of short chain fatty acids, such as: propionate, acetic acid, and butyrate. Butyrate is particularly important as a modulator of inflammation, with anti-inflammatory effects demonstrated in intestinal epithelial cells, macrophages, and leukocytes [28]. For example, butyrate acts as an important signaling molecule, influencing pathways that block NF-κB-induced increases in pro-inflammatory cytokines by a number of different mechanisms [11]. Butyrate also regulates the production of certain cytokines (e.g., TNF-α, IL-2, IL-6, IL-10), eicosanoids and chemokines from leukocytes, and affects their ability to migrate to sites of inflammation [14]. Furthermore, butyrate is an important inhibitor of histone deacetylase and has the potential to regulate expression of numerous genes, including those that encode important inflammatory mediators, i.e., nitric oxide (NO), IL-6 and IL-12 [29]. It also was recently shown to induce the differentiation and expansion of regulatory T cells not only in the colon [30] but also in the periphery [31], suggesting butyrate plays an important role in maintaining the balance between tolerance and hyper-reactivity. Finally, butyrate is a primary fuel source for colonocytes, and therefore helps maintain epithelial barriers in a number of different ways [32]. This evidence that butyrate also has important anti-inflammatory properties highlights the potential of using a carefully selected mixture of probiotic strains and compatible prebiotics (i.e. to formulate synbiotics) to maximize therapeutic efficacy [33].
Indirect effects: 3) Promote synthesis of antimicrobial peptides
Antimicrobial peptides (AMPs) are a group of small amphipathic peptides (e.g., defensins and cathelicidins) found in multicellular organisms such as plants and animals [34] and is produced by a wide variety of different cell types, including epithelial cells, leukocytes, intestinal Paneth cells [35] and keratinocytes [36, 37]. They were originally recognized and named for their role as potent broad-spectrum natural antimicrobials, providing a first line of defense at mucosal barriers, including those in the gastrointestinal and pulmonary tract [38]. Since their discovery, numerous additional functions have been determined, including important roles as immune signaling molecules that influence diverse functions, such as wound repair, expression of different chemokines and cytokines, recruitment of leukocytes, chemotaxis of mast cells, modulation of dendritic cell function and even modulation of adaptive immunity [39, 40]. In fact, AMPs are involved in such a widespread diversity of signaling that it is proposed they are “poorly named, antimicrobial peptides are multifunctional pillars around which the innate and adaptive immune response has evolved” [41]. Recently it was shown that these AMPs also act in prime inflammation resolution in the mucosa [42], suggesting they are part of an additional endogenous local pathway used to control an acute inflammatory response, bringing the associated inflamed tissue back to homeostasis. There are a number of different substances, including Vitamin D [43, 44], certain amino acids [45], butyrate [46], and probiotics [47], that are recognized as potent inducers of the synthesis and activity of AMPs.
Probiotics including Escherichia coli Nissle 1917, Lactobacillus fermentum PZ1162 and the mixture VSL# 3 were demonstrated to induce the secretion of AMP human beta-defensin -2 through pro-inflammatory pathways that involved the transcription factors NF-κB and AP-1 as well as MAPKs [47]. Several other types of AMPs including various bacteriocins are induced by various strains of probiotics (eg. L. salivarius DPC6005, L. salivarius UCC118, L. plantarum DDEN 11007, L. plantarum C11, L. lactis DPC3147) and demonstrated to have multiple roles in colonization, microbicidal activity as well as other signaling functions [48]. Although there is a need for further research, enhancing synthesis and secretion of AMPs might prove to be an additional indirect pathway whereby probiotics could help regulate an inflammatory response.
Direct effects: 1) Act as ligands for innate immune system receptors such as Toll-like receptors and nucleotide-binding oligomerization domain receptors
Probiotics also can have direct effects on the inflammatory response by acting as ligands for specific members of a large family of innate immune system receptors termed Toll-like receptors (TLRs), thereby influencing important signaling pathways, including NF-κB, mitogen-activated protein kinase (MAPK), phosphoinositide-3-kinase-protein kinase B/Akt (PI3K-PKB/Akt), and peroxisome proliferator-activated receptor gamma (PPARγ) [11]. TLR-2 and TLR-4 activation by probiotic microorganisms are recognized as particularly important receptors mediating the direct influence of probiotic and commensal bacteria on inflammatory signaling pathways [12, 49]; TLR-9 also is identified as an important receptor for mediating the anti-inflammatory effect of certain probiotic strains [12, 50].
Recent evidence suggests that some species of probiotics may release low molecular weight substances that can cross the epithelial cell membrane to interact with specific members of another large group of intracellular innate immune system receptors termed nucleotide-binding oligomerization domain receptors (NLRs) [12]. Triggering the downstream pathways associated with activation of NLRs represents another method whereby probiotics can affect inflammasome assembly and NF-κB associated signaling, thus the inflammatory response.
The ability of probiotics to influence NF-κ B and PPARγ signaling pathways would especially have widespread effects on inflammation, as these two transcription factors are recognized as critically important intracellular hubs at the intersection of many different pathways leading to production of numerous proinflammatory signals, including cytokines and chemokines. Activation of TLRs and NLRs also leads to assembly of inflammasomes (i.e., intracellular molecular platforms found in many cells that have a number of different regulatory functions, but are particularly responsible for activating the enzyme caspase-1 involved in the final maturation of the key pro-inflammatory cytokine IL-1β and IL-18) [51].
The effects of probiotics are strain specific, thus not all probiotics will have similar effects on each signaling pathway. A detailed summary of the current research on various probiotics and the specific signaling pathway they affect in different model systems has been published elsewhere [11] and will not be included in this paper. In sum, the binding of probiotics and commensal microflora to innate immune systems receptors such as TLRs and NLRs would help regulate major intracellular pathways that help maintain the homeostatic balance between pro-inflammatory and anti-inflammatory responses at mucosal surfaces.
It is important to note that some probiotics (e.g., Bifidobacterium lactis BB12, Bacteroides vulgatus) also stimulate NF-κB controlled signaling by a number of different mechanisms [11], suggesting they have pro-inflammatory effects. This pro-inflammatory effect of some probiotics could be beneficial, as suggested by current scientific evidence that a certain low level of inflammation could have physiological benefits including maintenance of epithelial barriers and ‘priming’ of immune system responses. Furthermore, the immunostimulatory phenotypes of commensal bacteria and probiotics might serve an important purpose of promoting host defense against pathogens.
Direct effects: 2) Influence maturation and development of important immune cells such as dendritic cells and T cells
In addition to their ability to affect intestinal epithelial cells and macrophages, probiotics also help influence the differentiation and function of a number of other immune cells associated with an inflammatory response, including dendritic cells as well as T cells (refer to [11, 12] for a diagrammatic representation). The ability of probiotics to affect the maturation, survival and function of dendritic cells [11] has widespread implications, because these professional antigen-presenting cells play key roles at mucosal surfaces in maintaining tolerance to commensal microbiota and innocuous food antigens. They also are an important source of cytokines that help promote differentiation of naive T cells into Th1, Th2, Th17 or T regulatory cells, thus the appropriate cell-mediated or humoral immune response [11]. Finally, different probiotic strains and species of beneficial microbes also influence the development and survival of different types of regulatory T cells, including Th3, Tr1, CD4+CD25+ regulatory, CD8+ suppressor and γδT cells [11], suggesting they can have a widespread role in maintaining intestinal homeostasis and the balance between oral tolerance and reactivity to ingested food antigens as well as to commensal microbes.
Direct effects: 3) Influence synthesis of the important regulatory cytokines IL-10 and TGF-β
Different populations of regulatory T cells exert their inflammation-modulating effect by secreting specific cytokines, including IL-10 (which strongly suppresses a Th-1 dominant pro-inflammatory response) or more regulatory cytokines such as TGF-β (which promotes development of regulatory T cells). Certain probiotic strains help regulate inflammation via their enhanced ability to influence the secretion of these important cytokines [11]; refer to [11, 12] for a diagrammatic representation. This effect has been demonstrated, in vitro [52, 53] and in vivo, in various animal models [54, 55, 56, 57] and clinical studies [58].
Clinically meaningful effects on inflammation?
There also are a limited number of studies demonstrating that supplementation with certain probiotics significantly reduced hs-CRP levels in adults that were healthy [59], pregnant [60], critically ill [61] or had inflammatory bowel disease [62] as well as other inflammatory disorders (e.g., ulcerative colitis, chronic fatigue syndrome and psoriasis) [63] and type 2 diabetes [64], suggesting that they could have a widespread anti-inflammatory effect that is clinically relevant.
Considerable heterogeneity exists in the clinical trial design published to date making it difficult to determine the exact dosing and strains of probiotics needed to ensure maximal therapeutic benefits in specific clinical conditions including inflammatory disease. For example, in the trials listed above there were major differences in study populations, types and daily dose of probiotics used, type of placebo, length of study as well as the delivery medium (Table 1).
Table 1: Summary of Clinical trials measuring effects of Probiotics on the inflammatory marker CRP
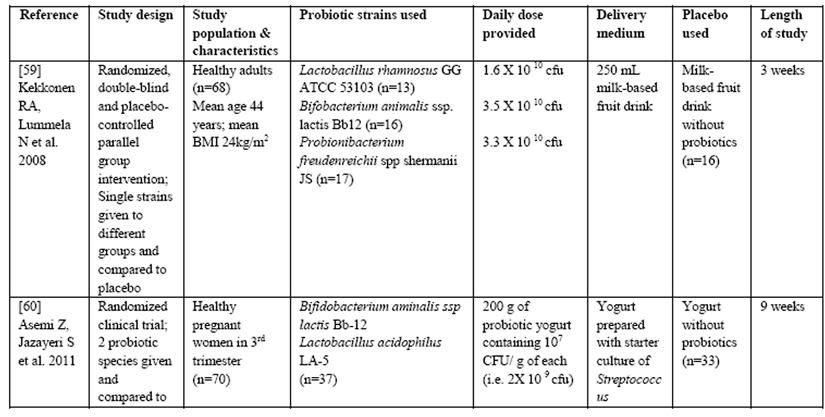
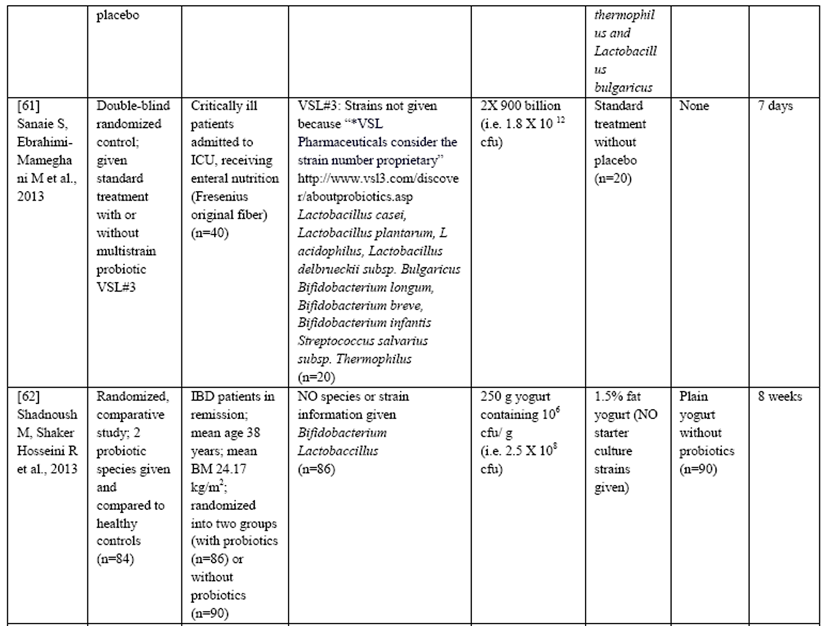
Furthermore, many studies do not list the strains of probiotics used in the intervention, even though it is becoming increasingly clear that not all strains have similar effects even if they are from the same species. All of these factors (and others) could potentially influence the ability of probiotics to control inflammation and therefore, it is difficult at this time to make definitive clinical recommendations. However, in general the range of doses used to alleviate symptoms of disease ranges from 100 million cfu/d for Bifidobacterium infantis 35624 to 300 to 450 billion cfu 3 times daily for VSL#3 (a mixture of 1 strain of Streptococcus thermophilus, 4 Lactobacillus species, and 3 Bifidobacterium species strains) in various clinical trials [65].
CONCLUSION
There is sufficient current scientific literature to support supplementation with probiotics as a beneficial addition to comprehensive treatment of inflammatory disease. Specific probiotic strains can help repair hyperpermeable epithelial barriers and therefore, indirectly regulate inflammation by shutting down potentially important sources of stimuli of chronic inflammation, including LPS. They also are important inducers of other molecules that play widespread roles in modulating inflammation, including butyrate and AMPs. Furthermore, specific strains and/or their components bind TLRs or NLRs to directly influence the activity of various important signaling pathways, influencing inflammation by promoting production of more suppressive and/or regulatory cytokines such as IL-10 and TGF-β. Using a combination of different well-researched probiotic strains to simultaneously modulate multiple signaling pathways could have a profound synergistic or additive effect on controlling and resolving inflammation in a timely fashion.
There is a need for additional preclinical and clinical research to further differentiate the optimum dose, posology, and exact strains of probiotics that have the most profound long-term effect on modulating the inflammatory response. Additional complexity in developing individualized inflammation regulating therapies using probiotics is created by the knowledge that different persons have unique endotypes of commensal microbiota as well as the recognition that a numerous factors, including age, genetics, use of certain medications, diet, geography and even psychological stress, can cause lasting shifts in commensal microbial ecology. Furthermore, the different types of diseases and even the phase the patient is in (especially in chronic relapsing diseases) will influence the quantity and quality of pro-inflammatory cytokines that need to be modulated by probiotics to produce clinically meaningful effects. Finally, it is unlikely that probiotics can act as a stand-alone therapy for treating inflammatory disease; therefore, it will be important to further delineate which therapies and medications can be used concomitantly to produce the safest and most effective clinical outcome.
List of abbreviations:
AMPs: antimicrobial peptides
γδT: gamma delta T cells
hsCRP: high-sensitivity C-reactive protein
IgA: immunoglobulin A
IL-10: interleukin-10
LPS: lipopolysaccharide (also termed endotoxin)
NO: nitric oxide
NF-κB: nuclear factor kappa B
NLRs: nucleotide-binding oligomerization domain receptors
MAPK: mitogen-activated protein kinase
PPAR-γ: peroxisome proliferator-activated receptor gamma
PI3K/PKB/Akt: phosphoinositide-3-kinase-protein kinase B
TGF-β: transforming growth factor beta
TLR: Toll-like receptor
TNF-α: tumor necrosis factor alpha
Competing interest:
Dr. Lescheid is a member of Medical Consultancy Group for Nutritional Fundamentals of Health, a company that formulates and sells Natural Health Products including probiotics. He also has lectured on this topic in the past, including lectures for this company.
Authors’ contributions:
DWL was the sole contributor to the conception, writing, revision and editing of this manuscript.
Author’s information:
DWL’s academic interest in probiotics was fostered by a Ph.D. in molecular biology (i.e. where microbes were used as vectors), a degree in Naturopathic medicine (i.e., where probiotics were taught as a potential part of a treatment plan for certain diseases) and by a five year position as an Assistant Professor teaching Microbiology and Infectious Disease. He also has seven years of clinical experience, using probiotics as safe and effective treatments for a variety of different conditions.
Acknowledgements:
None, there were no other contributors or sources of funding for this article.
REFERENCES:
Honad K, Littman DR:
The Microbiome in Infectious Disease and Inflammation.
Ann Rev Immunol 2012,30:758-795
Claros TG, Chang S, Gilliam EA, Maitra U, Deng H, Li L:
Causes and consequences of low grade endotoxemia and inflammatory diseases.
Front Biosci (Schol Ed.) 2013,5:754-765
Cani PD, Osto M, Geurts L, Everard A:
Involvement of gut microbiota in the development of low-grade inflammation and type 2 diabetes associated with obesity.
Gut Microbes 2012,3(4):279-288
Pussinen PJ, Havulinna AS, Lehto M, Sundvall J, Salomaa V:
Endotoxemia is associated with an increased risk of incident diabetes.
Diabetes Care 2011,34:392-397
Brun P, Castagliuolo I, Di Leo V, Buda A, Pinzani M, Palu G, Martines D:
Increased intestinal permeability in obese mice: new evidence on the pathogenesis of nonalcoholic steatohepatitis.
Am J Physiol Gastrointest Liver Physiol 2007,292:G518-525
Chang S, Li L: Metabolic endotoxemia:
A Novel Concept in Chronic Disease Pathology.
J Med Sci 2011,31(5) :191-209
Klein GL, Petschow BW, Shaw AL, Weaver E:
Gut barrier dysfunction and microbial translocation in cancer cachexia: a new therapeutic target. Curr Opin Support Palliat Care 2013,7(4) :361-367
Functional Foods in Health and Disease 2014; 4(7):299-311 Page 308 of 311
Wang B, Wu G, Zhou Z, Dai Z, Sun Y, Ji Y, Li W, Wang W, Liu C, Han F, Wu Z.
Glutamine and intestinal barrier function.
Amino Acids 2014 Jun 26. [Eub ahead of print]
Meracado J, Valenzano MC, Jeffers C, Sediak J, Cugliari MK et al.
Enhancement of Tight Junctional Barrier Function by Micronutrients: Compound Specific Effects on Permeability and Claudin Composition.
PLoS One 2013, 8(11):e78775
Mahmood A, Fitzgerald AJ, Marchbank T, Ntatsaki E, Murray D, Ghosh S, Playford RJ.
Zinc carnosine, a health food supplement that stabilizes small bowel integrity and stimulates gut repair processes.
Gut 2007, 56:168-175
Thomas CM, Versalovic J:
Probiotics-host communication. Modulation of signaling pathways in the intestine.
Gut Microbes 2010,1(3):148-163
Bermudez-Brito M, Plaza-Diaz J, Munoz-Quezada S, Gomez-Llorente C, Gil A:
Probiotic Mechanisms of Action.
Ann Nutr Metab 2012,61:160-174
Menard S, Candalh C, Bambou JC, Terpend K, Cerf-Bensussan N, Heyman M.
Lactic acid bacteria secrete metabolites retaining anti-inflammatory properties after intestinal transport.
Gut 2004,53:821-828
Heyman M, Terpend K, Menard S.
Effects of specific lactic acid bacteria on the intestinal permeability to macromolecules and the inflammatory condition.
Acta Paediatr Suppl 2005,94(449):34-36
Donato KA, Gareau M, Wang YJ, Sherman PM.
Lactobacillus rhamnosus GG attenuates interferon-{gamma} and tumor necrosis factor-a-induced barrier dysfunction and pro-inflammatory signalling.
Microbiology 2010,156:3288-3297
Ukena SN, Singh A, Dringenberg U, Engelhardt R, Seideler U, Hansen W, Bleich A, Bruder D, Franzke A, Rogler G, Suerbaum S, Buer J, Gunzer F, Westendorf AM.
Probiotic Escherichia coli Nissle 1917 inhibits leaky gut by enhancing mucosal integrity.
PLoS One 2007,12(2):e1308
Anderson RC, Cookson AL, McNabb WC, Park Z, McCann MJ, Kelly WJ, Roy NC.
Lactobacillus plantarum MB452 enhances the function of the intestinal barrier by increasing the expression levels of genes involved in tight junction formation.
BMC Microbiol 2010,10:316
Rao RK, Samak G.
Protection and Restitution of Gut Barrier by Probiotics: Nutritional and Clinical Implications.
Curr Nutr Food Sci 2013,9(2):99-107
Ohland CL, MacNaughton WK.
Probiotic bacteria and intestinal barrier function.
Am J Physiol Gastrointest Liver Physiol 2010;298:G807-G819
Khailova L, Frank DN, Dominguez JA, Wischmeyer PE.
Probiotic administration reduces mortality and improves intestinal epithelial homeostasis in experimental sepsis.
Anesthesiology 2013,119(1):166-177
Forsyth CB, Farhadi A, Jakate SM, Tang Y, Shaikh M, Keshavarzian A.
Lactobacillus GG treatment ameliorates alcohol-induced intestinal oxidative stress, gut leakiness, and liver injury in a rat model of alcoholic steatohepatitis.
Alcohol 2009,43(2):163-172
Sindhu KN, Sowmyanarayanan TV, Paul A, Baji S, Ajjampur SS, Priyadarshini S, Sarkar R, Balasubramanian KA, Wanke CA, Ward HD, Kang G.
Immune response and intestinal permeability in children with acute gastroenteritis treated with Lactobacillus rhamnosus
Functional Foods in Health and Disease 2014; 4(7):299-311 Page 309 of 311
GG (LGG): a randomized, double-blinded, placebo-controlled trial.
Clin Infect. Dis 2014, 58(8):1107-1115
Shing CM, Peake JM, Lim CL, Briskey D, Walsh NP, Fortes MB, Ahuja KD, Vitetta L.
Effects of probiotic supplementation on gastrointestinal permeability, inflammation and exercise performance in the heat.
Eur J Appl Physiol 2014,114(1):93-103
Lamprecht M, Bogner S, Schippinger G, Steinbauer K, Fankhauser F, Hallstroem S, Schuetz B, Greilberger JF.
Probiotic supplementation affects markers of intestinal barrier, oxidation, and inflammation in trained med; a randomized, double-blinded, placebo-controlled trial.
J Int Soc Sports Nutr 2012,9(1):45
Garcia Vilela E, De Lourdes De Abreu Ferrari M, Oswaldo Da Gama Torres H, Guerra Pinto A, Carolina Carneiro Aguirre A, Paiva Martins F, Marcos Andradr Goulart E, Sales Da Cunha A.
Influence of Saccharomyces boulardii on the intestinal permeability of patients with Crohn’s disease with remission.
Scand J Gastroenterol 2008,43(7):842-848
Rosenfeldt V, Benfeldt E, Valerius NH, Paerregaard A, Michaelsen KF.
Effect of probiotics on gastrointestinal symptoms and small intestinal permeability in children with atopic dermatitis.
J Pediatr 2004,145(5):612-616
Zeng J, Li YQ, Zuo XL, Zhen YB, Yang J, Lui CH.
Clinical trial: effect of lactic acid bacteria on mucosal barrier function in patients with diarrhea-predominant irritable bowel syndrome.
Aliment Pharmacol Ther 2008,28(8):994-1002
Vinolo MA, Rodrigues HG, Nachbar RT, Curi R.
Regulation of inflammation by Short Chain Fatty Acids.
Nutrients 2011,3:858-876
Chang PV, Hoa L, Offermanns S. Medzhitov R.
The microbial metabolite butyrate regulates intestinal macrophage function via histone deacetylase inhibition.
Proc Natl Acad Sci USA 2014,111(6):2247-2252
Furusawa Y, Obata Y, Fukuda S, Endo TA, Nakato G, Takahashi D, Nakanishi Y, Uetake C, Kato K, Takahashi M, Fukuda NN, Murakami S, Miyauchi E, Hino S, Atarashi K, Onawa S, Fujimura Y, Lockett T, Clarke JM, Topping DL, Tomita M, Hori S, Ohara O, Morita T, Koseki H, Kikichi J, Honda K, Hase K, Ohno H.
Commensal microbe-derived butyrate induces the differentiation of colonic regulatory T cells.
Nature 2013,504(7480):446-450
Arpaia N, Campbell C, Fan X, Dikiy S, van der Veeken J, deRoos P, Liu H, Cross JR, Pfeffer K, Coffer PJ, Rudensky AY.
Metabolites produced by commensal bacteria promote peripheral regulatory T-cell generation.
Nature 2013, 504(7480):451-455
Hamer HM, Jonkers D, Venema K, Vanhoutvin S, Troost FJ, Brummer RJ.
Review article: the role of butyrate on colonic function.
Aliment Pharmacol Ther 2008,27:104-119
Viera AT, Teixeira MM, Martins FS.
The role of probiotics and prebiotics in inducing gut immunity.
Frontiers Immunol 2013,445(4):1-12
Zasloff M.
Antimicrobial peptides of multicellular organisms.
Nature 2002,415:389-395
Martin E, Ganz T, Lehrer RI.
Defensins and other endogenous peptide antibiotics of vertebrates.
J Leukocyt Biol 1995,58(2):128-136
Gallo RL, Nakatsuji T.
Microbial symbiosis with the innate immune defense system of the skin. J Invest Dermatol 2011,131(10):1974-1980
Functional Foods in Health and Disease 2014; 4(7):299-311 Page 310 of 311
Schroder JM. The role of keratinocytes in defense against infection. Curr Opin Infect Dis 2010,23(2):106-110
Rogan MP, Geraghty P, Greene CM, O’Neill SJ, Taggart CC, McElvaney NG.
Antimicrobial proteins and polypeptides in pulmonary innate defense.
Respir Res 7:29
Elsbach P.
What is the real role of antimicrobial polypeptides that can mediate several other inflammatory responses?
J Clin Invest 200,111(11):1643-1645
Bowdish DM, Davidson DJ, Hancock RE.
Immunomodulatory properties of defensins and cathelicidins.
Curr Top Microbiol Immunol 2006,306:27-66
Schauber J, Gallo RL.
Expanding the roles of antimicrobial peptides in skin: alarming and arming keratinocytes.
J Invest Dermatol 2007,127(3):510-512
Campbell EL, Serhan CN, Colgan SP.
Antimicrobial Aspects of Inflammatory Resolution in the Mucosa: A Role for Proresolving mediators.
J Immunol 2011,187:3475-3481
Dombrowksi Y, Peric M, Koglin S, Ruzicka T, Schauber J.
Control of cutaneous antimicrobial peptides by vitamin D3.
Arch Dermatol Res 2010,302(6):401-408
Wang TT, Nestel FP, Bourdeau V, Nagai Y, Wang Q, Liao J, Tavera_Mednoza L, Lin R, Hanrahan JW, Mader S, White JH.
Cutting Edge: 1,25-Dihydroxyvitamin D3 is a Direct Inducer of Antimicrobial Peptide Gene Expression.
J Immunol 2004,173(5):2909-2912
Sherman H, Chapnik N, Froy O.
Albumin and amino acids upregulate the expression of human beta-defensin 1.
Mol Immunol 2006,43(10):1617-1623
Zasloff M.
Inducing endogenous antimicrobial peptides to battle infections.
Proc Natl Acad Sci USA. 2006,103(2):8913-8914
Schlee M, Harder J, Koten B, Stange EF, Wehkamp J, Fellermann K.
Probiotic lactobacilli and VSL#3 induce enterocyte beta-defensin 2.
Clin Exp Immunol 2008,151(3):528-535
Dobson A, Cotter PD, Ross RP, Hill C.
Bacteriocin Production: a Probiotic trait.
Appl Environ Microbiol 2012;78(1):1-6
Villena J, Kitazawa H.
Modulation of intestinal TLR4-inflammatory signaling pathways by probiotic microorganisms: lessons learned from Lactobacillus jensenii TL2937.
Front Immunol 2014; 4(512):1-12
Dogi CA, Weill F, Perdigon G.
Immune response of non-pathogenic Gram(+) and Gram (-) bacteria in inductive sites of the intestinal mucosa study of the pathway of signaling involved.
Immunobiology 2010,215(1):60-69
Schroder K, Tschopp J.
The inflammasomes.
Cell 2010,140:821-832
Smits HH, Engering A, van der Kleij, de Jong EC, Schipper K, van Capel TM, Zaat BA, Yazdanbakshs M, Wierenga EA, van Kooyk Y, Kapsenberg ML.
Selective probiotic bacteria induce IL-10-producing regulatory T cells in vitro by modulating dendritic cell function through dendritic cell-specific intercellular adhesion molecule 3-grabbing non-integrin.
J Allergy Clin Immunol 2005, 115(6):1260-7
Hseih PS, An Y, Tsai YC, Chen YC, Chunag CJ, Zeng CT, Want CT, An-Eri King V.
Potential of probiotic strains to modulate the inflammatory responses of epithelial and immune cells in vitro.
New Microbiol 2012,36(2):167-179
Oksaharuju A, Kooistra T, Kleemann R, van Duyvenvoorde W, Miettinen M, Lappalainen J, Lindstedt KA, Kovanen PT, Korpela R, Kekkonen RA.
Effects of probiotic Lactobacillus rhamnosus GG and Proprionibacterium freudenreichii ssp. shermani JS
Functional Foods in Health and Disease 2014; 4(7):299-311 Page 311 of 311
supplementation on intestinal and systemic markers of inflammation in ApoE*3Leiden mice consuming a high-fat diet. Br J Nutr 2013,119(1):77-85
Di Giacinto CD, Marinaro M, Sanchez M, Strober W, Boirivant M.
Probiotics ameliorate recurrent Th-1 mediated murine colitis by inducing IL-10 and IL-10-dependent TGF-b bearing regulatory cells.
J Immunol 2005,174:3237-3246
Calcinaro F, Dionisi S, Marinaro M, Candeloro P, Bonat V, Marzotti S, Corneli RB, Ferretti E, Gulino A, Grasso F, De Simone C, Di Mario U, Falorni A, Boirivant M, Dotta F.
Oral probiotic administration induces interleukin-10 production and prevents spontaneous autoimmune diabetes in the non-obese diabetic mouse.
Diabetologia 2005;48(8):1565-75
Jeon SG, Kayama H, Ueda Y, Takahashi T, Asahara T, Tsuji H, Tsuji NM, Kiyono H, Ma JS, Kusu T, Okumura R, Hara H, Yoshida H, Yamamoto M, Nomoto K, Takeda K.
Probiotic Bifidobacterium breve Induces IL-10-Producing Tr1 Cells in the Colon.
PLoS Pathog 2012,8(5):e1002714
Timmerman HM, Koning CJ, Mukder L, Rombouts FM, Beynen AC.
Monostrain, multistrain and multispecies probiotics—A comparison of functionality and efficacy.
Int J Food Microbiol 2004,96(3):219-233
Kekkonen RA, Lummela N, Karjalainen H, Latvala S, Tynkkynen S, Jarvenpaa S, Kautiainen H, Julkunen I, Vapaatalo H, Korpela R.
Probiotic intervention has strain-specific anti-inflammatory effects in healthy adults.
World J Gastroenterol 2008,14(13):2029-2036
Asemi Z, Jazayeri S, Najafi M, Samimi M, Mofid V, Shidfar F, Foroushani AR, Shahaboddin ME.
Effects of daily consumption of probiotic yogurt on inflammatory factors in pregnant women: a randomized controlled trial.
Pak J Biol Sci 2011,14(8):476-482
Sanaie S, Ebrahimi-Mameghani M, Mahmoodpoor A, Shadvar K, Golzari SE.
Effect of a Probiotic Preparation (VSL#3) on Cardiovascular Risk Parameters in Critically-Ill Patients.
J Cardiovasc Thorac Res 2013,5(2):67-70
Shadnoush M, Shaker Hosseini R, Mehrabi Y, Delpisheh A, Alipoor E, Faghfoori Z, Mohammadpour N, Zaringhalam Moghadam J.
Probiotic yogurt affects pro- and ant-inflammatory factors in patients with inflammatory bowel disease.
Iran J Pharm Res 2013,12(4):929-936
Groeger D, O’Mahony L, Murphy EF, Bourke JF, Dinan TG, Kiely B, Shanahan F, Quigley EM.
Bifidobacterium infantis 35624 modulates inflammatory processes beyond the gut.
Gut Microbes 2013,4(4):325-339
Asemi Z, Zare Z, Shakeri H Sabihi SS, Esmaillzaden A.
Effect of multispecies probiotic supplements on metabolic profiles, hs-CRP, and oxidative stress in patients with type 2 diabetes.
Ann Nutr Metabol 2013,63(1-2):1-9
Review Team, Guarner F, Khan AG, Garisch J, Eliakim R, Gangl A, Thomson A, Krabshius J, Lemair T, Kaufmann P, Andres de Paula J, Fedorak R, Shanahan F, Sanders Mary Ellen, Hania Szajewska, Siddartha Ramakrishna B, Karakan T, Kim N.
World Gastroenterology Organization Global Guidelines. Probiotics and Prebiotics. October 2011.
J Clin Gastroenterol 2012;46 (6):468-481
Return to the ACIDOPHILUS Page
Since 12-18-2014
|