FROM:
J Man Manip Ther 2022 (Nov 15); 1–9 ~ FULL TEXT
Lindsay M Gorrell, Andrew Sawatsky, W Brent Edwards, Walter Herzog
Integrative Spinal Research Group,
Department of Chiropractic Medicine,
University Hospital Balgrist and University of Zürich,
Zürich ,Switzerland.
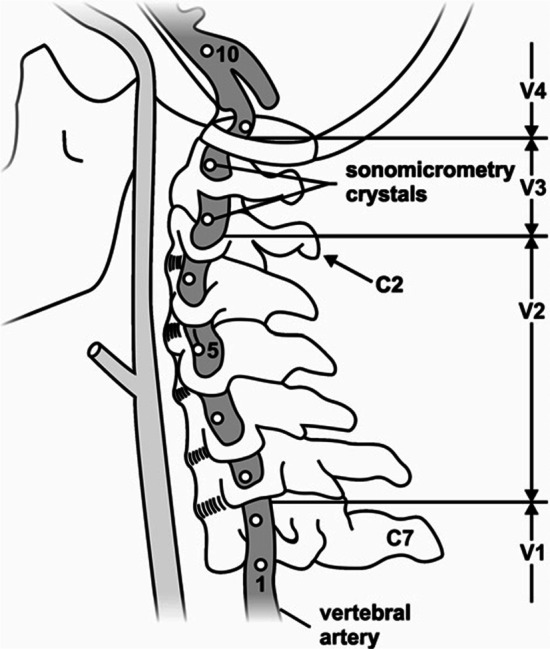
FROM:
J Electromyogr Kinesiol 2012
Background: The vertebral artery (VA) may be stretched and subsequently damaged during manual cervical spine manipulation. The objective of this study was to measure VA length changes that occur during cervical spine manipulation and to compare these to the VA failure length.
Methods: Piezoelectric ultrasound crystals were implanted along the length of the VA (C1 to C7) and were used to measure length changes during cervical spine manipulation of seven un-embalmed, post-rigor human cadavers. Arteries were then excised, and elongation from arbitrary in-situ head/neck positions to first force (0.1 N) was measured. Following this, VA were stretched (8.33 mm/s) to mechanical failure. Failure was defined as the instance when VA elongation resulted in a decrease in force.
Results: From arbitrary in-situ head/neck positions, the greatest average VA length change during spinal manipulation was [mean (range)] 5.1% (1.1 to 15.1%). From arbitrary in-situ head/neck positions, arteries were elongated on average 33.5% (4.6 to 84.6%) prior to first force occurrence and 51.3% (16.3 to 105.1%) to failure. Average failure forces were 3.4 N (1.4 to 9.7 N).
Conclusions: Measured in arbitrary in-situ head/neck positions, VA were slack. It appears that this slack must be taken up prior to VA experiencing tensile force. During cervical spine manipulations (using cervical spine extension and rotation), arterial length changes remained below that slack length, suggesting that VA elongated but were not stretched during the manipulation. However, in order to answer the question if cervical spine manipulation is safe from a mechanical perspective, the testing performed here needs to be repeated using a defined in-situ head/neck position and take into consideration other structures (e.g. carotid arteries).
Keywords: Spinal biomechanics; cerebrovascular accidents; spinal manipulation; stroke; vertebral artery dissection.
From the FULL TEXT Article:
Introduction
Neck pain has an annual global prevalence of 30 to 50% in the adult population and is a leading cause of musculoskeletal dysfunction worldwide. [1, 2] Cervical spine manipulation is an effective [3] and recommended [4–6] conservative treatment for neck pain. Further, in a recent scoping review reporting on utilization rates, neck pain is the second most common reason for patients to seek chiropractic care, accounting for 23% of all visits globally. In a recent review, it was reported that spinal manipulation is the most frequently used treatment modality during a chiropractic visit [median (IQR): 79.3% (55.4 to 91.3%) of treatment provided] with manual diversified-style techniques (i.e. manual contact of the involved vertebra with thrust into a rotation or lateral flexion direction) the most commonly used intervention [median (IQR): 65.5% (57.1 to 83.0%) proportion of use]. [7]
Certain cervical spine manipulations are associated with neck extension and rotation, leading to suggestions that these interventions stretch the vertebral artery (VA), thereby causing tissue damage. [8–12] Supporting this hypothesis, case studies suggesting a causal link between cervical spine manipulation and catastrophic adverse events, such as VA dissection and stroke, have been published. [13, 14] However, population-based studies suggest that there is no causal link between cervical spine manipulation and VA damage/stroke, rather there is a temporal (time) link. [15–23]
It is likely that this temporal association between cervical spine manipulation and VA dissection is based on the overlap of symptomatology for neck pain syndromes and arterial dissection. Specifically, the two most commonly reported symptoms of VA dissection are neck pain and headache which both mimic musculoskeletal dysfunctions. Thus, a patient experiencing a VA dissection may seek cervical spine manipulation for pain relief, believing that their symptoms are of mechanical origin. Additionally, it is possible that other structures (e.g. carotid arteries) may be responsible for similar symptoms. As such, consideration of the patient’s history and physical examination by the clinician cannot be understated when a decision to apply cervical spine manipulation is made. [24] These diverging reports in the literature (temporal vs. causal association), coupled with the routine use of cervical spine manipulation to treat neck pain, highlights the importance of directly investigating the biomechanical effects of cervical spine manipulation on the length changes and forces experienced by the VA.
In previous studies, the effects of cervical spine manipulation (using cervical spine extension and rotation and delivered at the end-range of segmental movement) delivered by chiropractors on the VA of cadaveric donors have been reported. [25–28] In these studies, piezoelectric ultrasound crystals measured VA length changes from a neutral, anatomical head/neck reference position, in either specific regions [27, 28] or, along the entire course of the vessel. [25, 26] The earliest work was conducted by Symons et al. who reported segmental length changes in the proximal (average: 1 to 8%) and distal (5 to 11%) regions of six VA during cervical spine manipulation delivered to C1/2, C3/4 and C6/7. [27] Following this, Wuest et al. measured length changes along the entire course of two VA and reported changes in the middle region (peak: 2 to 3%) during manipulation delivered to C2/3 and C4/5. [25]
These pilot data were later confirmed by Herzog et al. who reported length changes occurring in the proximal (range: 0 to 4%), middle (0 to 5%) and distal (0 to 13%) regions of eight VA during cervical spine manipulation applied to C1/2, C3/4 and C6/7. [26] In these studies, two different spinal manipulations were delivered with a second metacarpal contact. Both techniques involved the delivery of a high-velocity, low-amplitude thrust into either a rotation or lateral flexion direction (similar to the techniques reported in the current study). Most recently, Piper et al. investigated length changes in the distal region of six VA (range: ?15 to 18%) while also reporting head and neck displacements occurring during rotational cervical spine manipulation applied to the base of the occiput. [28]
However, a limitation of these studies was that spinal manipulations were not applied to all cervical vertebra (C1 to C7) on both sides of the neck while measuring VA length changes along the entire vessel. It should also be noted that the cervical spine manipulations delivered in these studies differed from those recommended in the most recent International Federation of Orthopaedic Manipulative Physical Therapists (IFOMPT) guidelines. [29] These guidelines recommend that rotation manipulations be delivered in the mid-range of cervical spine rotation, leveraging the coupled movements of lateral flexion and compression to target the intended level.
Mechanical testing has been used to investigate the biomechanical properties of human VA. [27, 30–32] For example, it has been reported that there is little change to the mechanical properties of extracranial arteries (VA included) up to the age of approximately 40 years. However, beyond 40 years, the authors reported an increased stiffness that was attributed to an increase in wall thickness. [31, 32] Johnson et al. reported on the mechanical properties of VA and observed that longitudinal strip samples failed at 16 to 20% elongation from zero engineering strain and that the tensile strength and load generated at peak force for the strip specimens (longitudinal direction) were lower than for radial ring samples. From these results, they concluded that the VA may be susceptible to tissue damage with longitudinal elongation (such as occurs during cervical spine manipulation). [30]
Symons et al. reported average VA failure length changes of 39 to 62% in the proximal and distal regions, which were measured with the head and neck in the neutral anatomical position. They concluded that arterial length changes experienced during cervical spine manipulation were almost an order of magnitude lower than the length changes required to mechanically disrupt the artery and thus, a single typical manipulative thrust was unlikely to mechanically disrupt the artery. [27] However, there have been no attempts to replicate these results or to quantify failure length changes occurring along the entire length of VA.
Therefore, the purpose of this study was to measure and compare VA lengths under four conditions:
i) in-situ, with the head and neck in arbitrary resting positions;
ii) at peak elongation (length change) during spinal manipulation applied bilaterally to all cervical vertebra;
iii) at the occurrence of first force with elongation (i.e. when slack was removed); and
iv) at mechanical failure.
Methods
Donor recruitment and preparation
Table 1
Figure 1
|
Recruitment took place between October 2016 and January 2020, utilizing the University of Calgary’s Body Donation Program. A convenience sample of seven un-embalmed, post-rigor human cadavers (fourteen VA) were obtained (Table 1). This study was approved by the University of Calgary Conjoint Health Research Ethics Board (REB16–0296). Blunt dissection of the anterior cervical region was performed to expose the VA. This included the removal of soft-tissues (e.g. larynx, pharynx, pre-vertebral muscles) and the mandible. Great care was taken to ensure that the minimum amount of tissue was removed to approximate the contributions of lateral and posterior soft tissues of the neck to the head and neck kinematics as closely as possible. The VA were instrumented with 2 mm piezoelectric ultrasound crystals bilaterally (Sonometrics Corporation, Canada). Eight crystals were secured into the lumen (via the lateral wall) of a single VA (Figure 1). Consistency in spacing (10 to 30 mm) and location was maintained across all donors and crystal insertion was performed as follows: at the mid-point between the subclavian artery and the C6 transverse foramen (1 crystal); at the mid-point between adjacent transverse foramen of C6-C2 (4 crystals); adjacent to the C2 and C1 transverse foramen respectively (2 crystals) and; distal to the C1 transverse foramen (1 crystal).
Data collection
Figure 2
|
Three chiropractic clinicians, with clinical experience ranging from 7 to 20 years, performed all cervical spine manipulations. Prior to the onset of data collection, two clinicians had previously delivered cervical spine manipulations to cadavers and the third was naïve. This clinician delivered several cervical spine manipulations to a cadaver prior to the commencement of data collection and reported feeling comfortable doing so. For each donor, data were collected from two clinicians, as it has been reported that there is inter-practitioner variability in the delivery of spinal manipulation. [33] The order of manipulation delivery was specified using the randomized number generator function in Matlab (vR2019b; Mathworks, USA). In this study, ipsilateral refers to the same side as the clinician’s primary contact while contralateral indicates the opposite side (i.e. for manipulation of C1 on the right, the right side of the donor’s neck was contacted (at the C1 level), and rotation of the head occurred to the left (contralateral)).
This convention was also used to describe the VA side (i.e. using the above example, the right artery would be ipsilateral and the left artery contralateral). Clinicians delivered two different manual supine diversified-style high-velocity, low-amplitude manipulations to each cervical vertebra and on both sides of the neck (C1 to C7) (Figure 2). For all manipulations, the articular process of the involved vertebra was targeted by the antero-lateral aspect of the proximal phalanx of the second digit of the clinician’s index finger. The pre-manipulative position for both procedures involved head and neck flexion, ipsilateral lateral flexion, and contralateral rotation. From this position, a rapid, controlled low-amplitude thrust was applied in a posterior-anterior (rotation) or medial and slightly inferior (lateral flexion) line of drive. [26]
For each trial, the force-time profile of the applied manipulation was recorded using a thin, flexible pressure pad (Novel, Germany, 100–200 Hz) enabling accurate identification of the peak of the manipulative thrust (defined as peak force). [27, 33] The pressure pad was placed between the clinician’s contact and the donor’s neck. To ensure synchronization between measurement systems, a 5 V electrical pulse was sent from the pressure measurement system to the remaining data collection computers at the beginning of each trial. VA length changes were captured using SonoSoft software (Sonometrics Corporation, Canada, 260–557 Hz). This method has been demonstrated to have a spatial resolution of 16 µm. [23] Prior to every test, the in-situ length of the artery was measured with the head in an arbitrary resting position. Prior to, and as necessary throughout data collection, arteries were perfused with ultrasonographic gel to best mimic realistic in-vivo shape and promote ultrasound signal transmission. Data from eight analogue channels were collected simultaneously for each testing procedure, recording arterial length changes during movement.
Immediately following cervical spine manipulation testing, the entire artery was carefully harvested using blunt dissection. The arteries were stored in a physiological saline solution prior to mechanical failure testing which took place within 24 hr post-excision. When it was not possible to immediately perform failure testing, arteries were refrigerated at 4°C and allowed to return to room temperature prior to data collection. A phosphate buffered saline solution was used to keep the tissue moist throughout testing.
Mechanical testing was performed using displacement control on an Instron Electropuls E3000 materials testing machine (Instron, USA). Arteries were elongated to the length of first force occurrence (0.1 N, as measured by a 100 N force transducer (Omega Engineering Inc., USA)), hereafter referred to as first force length, and ultrasound gel was introduced into the lumen to promote signal transmission. Note: first force refers to the longitudinal force measured in the VA, not the ‘first force’ that may be experienced by the clinician during cervical spine manipulation. Following preconditioning of the artery (1,000 cycles at 6% length change), the artery was infused with ultrasound gel and immediately stretched to mechanical failure at 8.33 mm/sec. Failure was defined as the first point when stretching of the artery resulted in a decrease in force (first negative stiffness). During mechanical testing, arterial length changes were measured as described above using the ultrasound crystals that were implanted prior to cervical spine manipulation. Forces were recorded with a personal laptop computer (Windaq, DATAQ Instruments Inc., USA, 400 Hz).
Data analysis
All data were exported to Excel (Microsoft, USA) for analysis. Segmental lengths between adjacent ultrasound crystals were summed to give the overall length of the artery. VA length change was calculated as:
D?=?(L1 - L0)/L0 ,
where L0 was the in-situ length of the artery when the head and neck were resting on the gurney in an arbitrary position, and L1 was the length of the artery at: i) peak force occurrence during the manipulative thrust; or ii) the point of failure.
Statistical analysis
Descriptive statistics [mean (range)] were used to report length change and force data for cervical spine manipulation and mechanical failure testing. Differences in length changes were calculated using Wilcoxson Signed Rank Testing Exact method (SPSS, version 26, IBM, USA). Statistical significance was set at p < 0.05.
Results
Data are presented below for 518 cervical spine manipulation trials and 14 mechanical failure tests.
Cervical spine manipulation
VA length changes were generally greater in the contralateral than the ipsilateral artery for both rotation (p = 0.016) and lateral flexion (p = 0.016) manipulations. Ipsilateral VA length changes were generally lower when using the lateral flexion technique compared to the rotation technique (p = 0.031), but there was no difference between the two techniques for the contralateral VA (p = 0.813). Average peak length changes were greatest in the contralateral VA for lateral flexion manipulations applied to the C5 vertebra [mean (range)] 5.1% (range: 1.1 to 15.1%) and smallest for the ipsilateral VA for lateral flexion manipulations applied to the C1 vertebra 1.1% (0.0 to 2.9%) (Table 2).
Mechanical failure testing
VA had to be elongated on average by 33.5% (range 4.6 to 84.6%) from their arbitrary in-situ lengths before slack was taken up and first force was measured, indicating that the arteries carried no load and were never stretched, but merely elongated, during all cervical spine manipulations. VA failure occurred at an average force of 3.4 N (1.4 to 9.7 N) and an average length change of 51.3% (16.3 to 105.1%) from the arbitrary in-situ lengths (Table 3 & Figure 3).
Discussion
The purpose of this study was to measure and compare VA length changes under four conditions: i) in-situ, with the head and neck in an arbitrary resting position; ii) at peak elongation (length change) during spinal manipulation applied bilaterally to all cervical vertebra; iii) at the occurrence of first force (i.e. when slack was removed from the VA when elongating it from its in-situ length); and iv) at the occurrence of mechanical failure.
The primary findings of this study were that: (i) the average peak length changes during cervical spine manipulations (Table 2) were much smaller than either the average length change at the occurrence of first force or the average length change at mechanical failure (Table 3 & Figure 2); (ii) of the 518 cervical spine manipulations applied in this study, on only two occasions, in a single artery, did length change during the manipulative thrust (5.6%) exceed that artery’s length change at first force occurrence (4.6%) and never came close to the failure length changes (16.3%); and(iii) as a consequence of points (i) and (ii) despite the variability in the length changes in the different specimens, the peak length changes during cervical spine manipulation do not generally reach or exceed the length change at first force for VA.
The length change values obtained in this study agree with previously reported VA length changes during cervical spine manipulation [25-28] and mechanical failure testing [27], despite using an arbitrary and changing reference length (head resting on the gurney vs. head in the neutral anatomical position [25-28]). Differences in reference length between the arbitrary resting (head on gurney) and neutral (head in hands) were calculated for a subset of the data (range of motion and not reported here) and VA length was (average?±?SD) 1.0 ± 1.5% shorter in the neutral compared to arbitrary resting position. The maximum difference between the two positions was 6.4% shorter in the neutral position. To place this in context using lengths commonly seen in this type of experiment and an extreme difference in lengths of 6.5% – given a VA length of 100 mm, with an elongation during cervical spine manipulation of 3 mm, the length change would be equal to 3%. For the same cervical spine manipulation, if the length was 6.5% shorter in the neutral vs. the resting position (i.e. 93.5?mm), the resulting length change would be 10.2% and if 6.5% longer (i.e. 106.5 mm), ?3.3%. Thus, by using arbitrary resting positions, the actual values of strain may be under- or overestimated on average by about 10% for an average VA strain of 3%.
The VA was slack in its resting, in-situ length and required an average of 33.5% elongation prior to first force occurrence, suggesting that the VA is not stretched during cervical spinal manipulation, but merely some of the slack naturally present is taken up during the procedure. This finding agrees with previous results that cervical spine manipulations produced peak length changes of about 6% [27] (measured from the neutral anatomical head and neck position), and these length changes were not associated with measurable force upon mechanical failure testing. [33, 35] It has been suggested that the VA might be excessively stretched during cervical spinal manipulation, and that these stretches might cause structural damage to the VA. The results of this study, in conjunction with previously published results that VA length changes during spinal manipulation are about half of those experienced during normally achievable head and neck movements [27, 33, 35], suggest that the VA cannot be mechanically damaged by elongation that occurs during cervical spinal manipulation (using cervical spine extension and rotation).
While failure length changes for the VA obtained in this study were similar to those reported previously [27, 30], the average failure forces reported here (3.4 N) were approximately half of those previously measured, which were on average 8.2 to 8.8 N. [27] The reasons for this difference are not known but could be due to a variety of factors. Firstly, in the current study, there were more than double the number of ultrasound crystals implanted into the VA. Implantation of each crystal causes a small amount of damage to the vessel, which may reduce the forces measured for a given amount of total length change. Secondly, ultrasound gel was injected into the VA throughout testing to enhance signal transmission and to preserve the in-vivo shape of the blood-filled artery. While this was done only when necessary and using a small-gauge needle to minimize disruption to the arterial wall, damage may have occurred that reduced the forces for a given total tissue length change magnitude.
Thirdly, it is possible that during dissection and instrumentation of the donor, there was disruption to the transforaminal fascial bands anchoring the VA to the transverse foramen. While all care was taken to prevent this occurring, it is unknown if and/or to what extent this occurred and what effect this may have had on bending loads at the foraminal entrance and exit points (which was not quantified). Finally, the lower failure forces seen in the current study may be the result of the viscoelastic decrease in force that occurred during the preconditioning performed prior to the failure tests. Since failure testing was performed following a preconditioning protocol when VA forces had reached a steady-state, with no pause for force recovery, a part of the observed decrease in failure force compared to literature values may be accounted for by the lack of tissue recovery.
This result is supported by the fact that failure length changes in the only comparable study [27] were similar to those observed here and that in the previous study, failure testing was not preceded by cyclic preconditioning protocols and steady-state force that would have depressed the force-length change relationship.
Furthermore, failure forces for the VA varied greatly in our study (range: 1.4 to 9.7 N) and in previously published investigations (mean ± SD: 8.2 ± .4 N and 8.8 ± 5.4 N for the distal and proximal segments of the VA, respectively [27]), and thus, our results may just be a matter of random variation. Nevertheless, it is likely that the failure forces reported here underestimate those occurring in an un-instrumented, intact VA, and that most of this underestimation may be accounted for by tissue damage during instrumentation and testing and the force relaxation that occurred in the preconditioning protocol immediately preceding the failure testing.
Limitations
The results of this study should be interpreted with consideration of the following limitations. Firstly, the designation of first force in this study referred to the measurement (via a material testing machine) of passive longitudinal force and did not consider other stresses or strains (active and passive) present in-vivo. However, it is currently impossible to measure such forces except via the use of specialized equipment. Furthermore, in a clinical setting, and in our test scenario, it is not possible to determine first force onset on the VA, first and foremost because other structures offer much more resistance to neck motion than the VA, and secondly, our analysis revealed that the VA is never stretched during any of the cervical spine manipulations, thus any force felt by a clinician must originate from structures other than the VA.
Secondly, as mechanical failure testing was only conducted in the longitudinal direction, information concerning circumferential and radial length changes were not investigated. However, it has been suggested that longitudinal length change places VA at the highest risk of damage [30, 36] and we are confident that the current experimental set-up approximated the in-vivo mechanical environment during cervical spine manipulation. Moreover, no attempt was made to replicate the chemical environment. As such, these results are limited to mechanical elongation of the VA and conclusions regarding other cervical artery disorders (e.g. embolism) cannot be made.
Additionally, no information was recorded regarding the elongation and/or forces experienced by the different layers of the VA wall (i.e. intima, media and externa) and it is unknown if these elongations/forces were uniform across all layers. However, as our results indicate that there was no longitudinal force applied during cervical spine manipulation, it is unlikely that there would be any longitudinal force in any VA layer. Indeed, the only way to measure these forces would be to excise the vessel and perform experiments in a material testing system (e.g. Instron). Typically, such systems measure total force, independent of which layer the force is occurring in.
Another consideration is the generalizability of the current results to the adult population receiving cervical spine manipulation. Specifically, all data were collected on human cadaveric donors of advanced age (average ± SD; 85 ± 11 years) with pre-existing health conditions, many of which were related to cardiovascular disease, contraindicating them for cervical spine manipulation in a clinical situation. Further, with increasing age, arterial walls typically become thicker, less distensible, stiffer [31, 32] and the tethering force decreases [37]. As such, the mechanical properties described in this study may not directly reflect those seen in younger, healthier arteries. However, it is likely that the VA failure force in a human cadaver and live human are similar, as collagen (a biochemically stable molecule [38]) is the main load-bearing structure in the vessel [39] and the mechanical properties of human arterial tissues are similar in cadavers and live humans. [40]
While not investigated in this study, other factors for consideration regarding differences between the study cohort and clinical cohort in which cervical spine manipulation (including rotational procedures) would typically be applied possibly include: i) the presence/absence of any discogenic instability; ii) spondylotic displacement of the VA by uncinate process hypertrophy; and iii) arthritic enlargement of the superior facet. These are salient points as VA dissection most commonly occurs in outwardly healthy adults younger than 45 years of age. [15]
Furthermore, arterial tissue is viscoelastic [41, 42] and becomes stiffer with increasing loading rate. [36, 42] Thus, it is possible that VA may have failed at higher forces if the stretch to failure had been applied at faster speeds. However, Symons et al. reported that there was no difference during VA failure testing between elongations randomly performed at 5 mm/min and 500 mm/min and that the faster loading rate approximate those occurring during cervical spine manipulation. [27] Finally, as viscoelastic tissues display hysteresis (i.e. the length of the specimen depends on the loading history), it is possible that the VA length changes reported here may be different to those occurring in a clinical encounter.
The repeated cervical spine manipulations, and the preconditioning protocol implemented prior to failure testing represent the biomechanical loading history. However, it is reasonable to assume that the results presented here are a ‘worst case scenario’ as likely the number of cervical spine manipulations delivered to an individual in a clinical scenario would be less than in our protocol, and if there was micro-damage to the VA wall, there would be time between treatment sessions for the damage to heal.
However, since no segment of the VA was ever stretched during cervical spine manipulation, but merely elongated (i.e. some of the natural slack of the VA was taken up), considerations of viscoelasticity only apply to the final failure testing experiments but not to the cervical spine treatments performed on the specimens.
Conclusions
Measured from arbitrary in-situ positions of the head/neck, VA had a certain amount of slack. At peak lengths during cervical spine manipulation (using cervical spine extension and rotation), longitudinal VA length changes remained below that slack length, suggesting that VA elongated but were not stretched during the intervention. However, in order to answer the question if cervical spine manipulation is safe from a mechanical perspective, further testing using a defined in-situ head/neck reference position for all VA length change measurements, allowing for comparisons between treatments and across donor specimens should be conducted. To answer this question unequivocally, VA length changes should also be measured during cervical spine manipulation delivered to live patients and take into consideration other structures (e.g. carotid arteries)
Acknowledgements
The authors would like to acknowledge Drs. Ryan Carter and Bruce Symons for their clinical expertise delivering the manual procedures during testing.
Funding Statement
This work was supported by the Canadian Chiropractic Research Foundation and the Alberta College and Association of Chiropractors. There was no involvement of either funding body in the study design, collection, analysis and interpretation of data, writing of the manuscript, and/or decision to submit the article for publication.
Disclosure statement
No potential conflict of interest was reported by the author(s).
Author contributions
Lindsay Gorrell: Conceptualization, Methodology, Formal Analysis, Investigation, Writing – Original Draft and Writing - Review and Editing.
Andrew Sawatsky: Investigation, Writing – Review and Editing.
Brent Edwards: Resources, Writing – Review and Editing.
Walter Herzog: Conceptualization, Methodology, Resources, Writing – Review and Editing.
REFERENCES:
Martin BI, Deyo RA, Mirza SK, et al.
Expenditures and Health Status Among Adults With Back and Neck Problems
JAMA 2008 (Feb 13); 299 (6): 656–664
James SL, Abate D, Abate KH, et al.
Global, regional, and national incidence, prevalence, and years lived
with disability for 354 diseases and injuries for 195 countries
and territories, 1990–2017: a systematic analysis for
the global burden of disease study 2017.
Lancet. 2018. Nov 10;392(10159):1789–1858.
Bronfort G, Haas M, Evans R, et al.
Effectiveness of Manual Therapies:
The UK Evidence Report
Chiropractic & Osteopathy 2010 (Feb 25); 18 (1): 3
A.E. Bussières, G. Stewart, F. Al-Zoubi, P. Decina, M. Descarreaux, et al
The Treatment of Neck Pain-Associated Disorders
and Whiplash-Associated Disorders:
A Clinical Practice Guideline
J Manipulative Physiol Ther. 2016 (Oct); 39 (8): 523–564
Whalen W, Farabaugh RJ, Hawk C, et al.
Best-Practice Recommendations for Chiropractic Management
of Patients With Neck Pain
J Manipulative Physiol Ther. 2019 (Nov); 42 (9): 635–650
Bier JD, Scholten-Peeters WGM, Staal JB, et al.
Clinical practice guideline for physical therapy assessment
and treatment in patients with nonspecific neck pain.
Phys Ther. 2018. Mar 1;98(3):162–171.
Beliveau PJH, Wong JJ, Sutton DA, et al.
The Chiropractic Profession: A Scoping Review of
Utilization Rates, Reasons for Seeking Care,
Patient Profiles, and Care Provided
Chiropractic & Manual Therapies 2017 (Nov 22); 25: 35
Di Fabio RP.
Manipulation of the cervical spine: risks and benefits.
Phys Ther. 1999. Jan;79(1):50–65.
Frisoni GB, Anzola GP.
Vertebrobasilar ischemia after neck motion.
Stroke. 1991. Nov;22(11):1452–1460.
Assendelft WJ, Bouter LM, Knipschild PG.
Complications of spinal manipulation:
a comprehensive review of the literature.
J Fam Pract. 1996. May;42(5):475–480.
Albuquerque FC, Hu YC, Dashti SR, et al.
Craniocervical arterial dissections as sequelae of chiropractic manipulation:
patterns of injury and management.
J Neurosurg. 2011. Dec;115(6):1197–1205.
Ernst E.
Adverse effects of spinal manipulation: a systematic review.
J R Soc Med. 2007. Jul;100(7):330–338.
Jones J, Jones C, Nugent K.
Vertebral artery dissection after a chiropractor neck manipulation.
Proc Bayl Univ Med Cent. 2015. Jan;28(1):88–90.
Ernst E.
Deaths after chiropractic: a review of published cases.
Int J Clin Pract. 2010. Jul;64(8):1162–1165.
J. Biller, R.L. Sacco, F.C. Albuquerque, B.M. et al
Cervical Arterial Dissections and Association With Cervical Manipulative
Therapy A Statement for Healthcare Professionals From the
American Heart Association/American Stroke Association
Stroke. 2014 (Oct); 45 (10): 3155–3174
Boyle E, Côté P, Grier AR, et al.
Examining Vertebrobasilar Artery Stroke in Two Canadian Provinces
Spine (Phila Pa 1976). 2008 (Feb 15); 33 (4 Suppl): S170–175 .
J.D. Cassidy, E. Boyle, P. Côté, Y. He, S. Hogg-Johnson, F.L.
Risk of Vertebrobasilar Stroke and Chiropractic Care:
Results of a Population-based Case-control
and Case-crossover Study
Spine (Phila Pa 1976) 2008 (Feb 15); 33 (4 Suppl): S176–183
Choi S, Boyle E, Côté P, et al.
A Population-Based Case-Series of Ontario Patients
Who Develop a Vertebrobasilar Artery Stroke
After Seeing a Chiropractor
J Manipulative Physiol Ther 2011 (Jan); 34 (1): 15–22
T.M. Kosloff, D. Elton, J. Tao, W.M. Bannister
Chiropractic Care and the Risk of Vertebrobasilar Stroke:
Results of a Case-control Study in U.S. Commercial
and Medicare Advantage Populations
Chiropractic & Manual Therapies 2015 (Jun 16); 23: 19
Lee VH, Brown RD, Mandrekar JN, et al.
Incidence and outcome of cervical artery dissection:
a population-based study.
Neurology. 2006. Nov 28;67(10):1809–1812.
Puentedura EJ, March J, Anders J, et al.
Safety of cervical spine manipulation: are adverse events preventable
and are manipulations being performed appropriately?
A review of 134 case reports.
J Man Manip Ther. 2012. May;20(2):66–74.
D.M. Rothwell, S.J. Bondy, J.I. Williams
Chiropractic Manipulation and Stroke:
a Population-based Case-control Study
Stroke 2001 (Sep); 32 (9): 2207—2208
Smith W, Johnston S, Skalabrin E, et al.
Spinal manipulative therapy is an independent risk factor
for vertebral artery dissection.
Neurology. 2003. May 13;60(9):1424–1428.
Rushton A, Rivett D, Carlesso L, et al.
International framework for examination of the cervical region for
potential of cervical arterial dysfunction prior to
orthopaedic manual therapy intervention.
Man Ther. 2014. Jun;19(3):222–228.
Wuest S, Symons B, Leonard T, et al.
Preliminary report: biomechanics of vertebral artery segments
C1-C6 during cervical spinal manipulation.
J Manipulative Physiol Ther. 2010. May;33(4):273–278.
W. Herzog, T.R. Leonard, B. Symons, C. Tang, S. Wuest
Vertebral Artery Strains During High-speed,
Low amplitude Cervical Spinal Manipulation
J Electromyo and Kinesio 2012 (Oct); 22 (5): 740–746
Symons BP, Leonard T, Herzog W.
Internal Forces Sustained by the Vertebral Artery
During Spinal Manipulative Therapy
J Manipulative Physiol Ther 2002 (Oct); 25 (8): 504–510
Piper SL, Howarth SJ, Triano J, et al.
Quantifying Strain in the Vertebral Artery With Simultaneous Motion
Analysis of the Head and Neck: A Preliminary Investigation
Clin Biomech (Bristol, Avon) 2014 (Dec); 29 (10): 1099–1107
Rushton A, Carlesso LC, Flynn T, et al.
International framework for examination of the cervical region for potential
of vascular pathologies of the neck prior to orthopaedic manual
therapy (OMT)
Intervention:
international IFOMPT cervical framework. International Federation
of Orthopaedic Manipulative Physical Therapists (IFOMPT). 2020
[Available at: https://www.ifompt.org/site/ifompt/IFOMPT
%20cervical%20framework%20final%202020.pdf]
Johnson CP, How T, Scraggs M, et al.
A biomechanical study of the human vertebral artery
with implications for fatal arterial injury.
Forensic Sci Int. 2000. Apr 10;109(3):169–182.
Hayashi K, Handa H, Nagasawa S, et al.
Stiffness and elastic behavior of human intracranial and extracranial arteries.
J Biomech. 1980. Jan 1;13(2):175–184.
Nagasawa S, Handa H, Okumura A, et al.
Mechanical properties of human cerebral arteries.
Part 1: effects of age and vascular smooth muscle activation.
Surg Neurol. 1979. Oct;12(4):297–304.
Herzog W.
The Biomechanics of Spinal Manipulation
J Bodyw Mov Ther. 2010 (Jul); 14 (3): 280–286
Gorrell LM, Kuntze G, Ronsky JL, et al.
Kinematics of the Head and Associated Vertebral Artery Length Changes
During High-velocity, Low-amplitude Cervical Spine Manipulation
Chiropractic & Manual Therapies 2022 (Jun 1); 30 (1): 28
Herzog W, Symons B.
The Mechanics of Neck Manipulation With
Special Consideration of the Vertebral Artery
J Can Chiropr Assoc. 2002 (Sep); 46 (3): 134–136
Mann T, Refshauge KM.
Causes of complications from cervical spine manipulation.
Aust J Physiother. 2001. Jan 1;47(4):255–266.
Thubrikar MJ.
Vascular mechanics and pathology. 1st ed.
USA: Springer, USA; 2007.
Shoulders MD, Raines RT.
Collagen structure and stability.
Annu Rev Biochem. 2009;78(1):929–958.
Piffer CR, Zorzetto NL.
Microscopy anatomy of the vertebral artery in the
suboccipital and intracranial segments.
Anat Anz. 1980;147(4):382–388.
Arturo Larco JL, Madhani SI, Liu Y, et al.
Human “live cadaver” neurovascular model for proximal and
distal mechanical thrombectomy in stroke.
J Neurointerv Surg. 2022. Apr 13;018686. neurintsurg-2022-. DOI: 10.1136/neurintsurg-2022-018686
Austin N, DiFrancesco LM, Herzog W.
Microstructural Damage in Arterial Tissue
Exposed to Repeated Tensile Strains
J Manipulative Physiol Ther 2010 (Jan); 33 (1): 14–19
Westerhof N, Noordergraaf A.
Arterial viscoelasticity: a generalized model: effect on
input impedance and wave travel in the systematic tree.
J Biomech. 1970. May 1;3(3):357–379.
Return to STROKE AND CHIROPRACTIC
Since 5-18-2023
|